Space-based WMO Integrated Global Observing System
Global Planning
This section describes the current global planning for operational geostationary satellites and Low-Earth Orbit satellites, as developed in consultation among WMO and satellite operators within the Coordination Group for Meteorological Satellites (CGMS). It includes a nominal configuration and a global contingency plan.
Observation requirements and related observing capabilities are kept under review as part of the Rolling Review of Requirements (RRR) process and a Gap Analysis of the space-based GOS is regularly performed. Gap analyses are maintained in the on-line database tool OSCAR (Observing System Capabilities Analysis and Review)
WMO has developed the "Vision for the WMO Integrated Global Observing System in 2040" that provides a long-term goal to foster the development of the WIGOS and meet the challenges of Earth system observation. The vision (addressing both surface- and space-based observation) was adopted by the 18th World Meteorological Congress, held in June 2019 (Resolution 38(Cg-18)).
Global planning for operational geostationary satellites
The nominal constellation of operational geostationary satellites includes 6 spacecraft to ensure full coverage from 50°S to 50°N with a zenith angle lower than 70°.
Nominal geostationary locations agreed among CGMS satellite operators are:
Region |
Nominal operator |
Nominal operational locations |
North, Central and South America (GOES-West) |
USA (NOAA) |
135° W |
East Pacific (GOES-East) |
USA (NOAA) |
75° W |
Europe & Africa |
EUMETSAT |
0° |
Indian Ocean |
Russian Federation (Roshydromet) |
76° E |
Asia |
China (CMA) |
105° E |
West Pacific |
Japan (JMA) |
140° E |
Note that different locations may be used, depending on operational constraints and spacecraft availability.
While space-based observation increasingly involves Low-Earth Orbit satellites (either from operational or from R&D environmental missions) that provide essential input to numerical modelling, the constellation of operational meteorological geostationary satellites remains the backbone of permanent and near-global monitoring of the weather situation. Infrared composite imagery as shown above is produced on an operational basis with data from GOES-West and GOES-East (NOAA, USA), Meteosat and Meteosat/IODC (EUMETSAT) and Himawari (JMA, Japan). Additional satellites like the FY-4 series (CMA, China) contribute to strengthen the system and ensure its operational continuity.
Global planning for Low Earth Orbit sun-synchronous operational missions
The new baseline for the core LEO constellation is to be deployed over three orbital planes around 13:30, 17:30 and 21:30 Equatorial Crossing Time (ECT) in Local Solar Time (LST). This should ensure regular sampling of the atmosphere avoiding too large a temporal gap around dawn and dusk, in order to satisfy as far as possible the observing cycle requirements from NWP and climate monitoring as concerns atmospheric temperature and humidity profiles. In addition, in-orbit redundancy should be available around these orbital planes, to the extent possible.
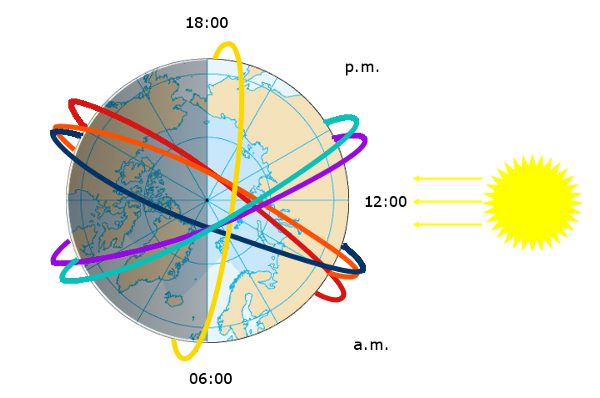
Global Contingency Planning
The CGMS members have agreed a Global Contingency Plan among satellite operators and they keep this plan under regular review.
Continuity of the geostationary imaging service is essential for meteorological operations in support of severe weather warning, and Numerical Prediction in general. Such continuity is ensured through maintaining additional satellites in stand-by position for in-orbit back-up, and through bilateral contingency agreements among neighbouring geostationary satellite operators. Through such agreements, operators agree that, in case of major satellite failure encountered by an operator, and if another operator has a spare satellite available, the spare satellite could be relocated to replace the failing satellite on a temporary basis until a nominal configuration can be recovered through the launch of a new satellite.
Continuity of low-Earth orbit services is achieved through redundancy on the am and pm orbital missions.
Download the CGMS Global Contingency Plan.
Vision for WIGOS in 2040 (Summary of the space-based component)
Download the description of the WMO "Vision for WIGOS in 2040".
Compared to their current requirements, it is expected that by 2040, users will require:
(a) Higher resolution observations and better temporal and spatial sampling/coverage;
(b) Improved data quality and consistent characterization of uncertainty;
(c) Novel data types, allowing insight into hitherto poorly understood Earth system processes, including space weather;
(d) Efficient and interoperable data representation and dissemination methodology given the anticipated continued growth in data volumes.
Even in the near term, certain additional observations using existing technology are required to address immediate needs and gaps in several specific WMO application areas. These include:
(a) Atmospheric composition: limb sounding for the upper troposphere and stratosphere/mesosphere, nadir sounding using short-wave infrared (SWIR) spectrometry, trace gas light detection and ranging (lidar);
(b) Hydrology and cryosphere: laser and radar altimetry, visible multifrequency synthetic aperture radar (SAR) and passive microwave imagery;
(c) Cloud phase detection for NWP: sub-mm imagery;
(d) Aerosol and radiation budget: multiangle, multipolarization radiometry; lidar;
(e) Wind: lidar and hyperspectral capabilities;
(f) Solar wind/solar eruptions: solar wind monitor, magnetometer, energetic particle sensor, solar extreme ultraviolet (EUV) imagery, heliospheric imagery (at L5) and in situ energetic particle flux (at L1).
It is expected that rapid progress in remote sensing technology will lead to the development of sensors with higher signal sensitivity, which will result in potentially higher spatial, temporal, spectral and/or radiometric resolution. New types of sensors will ensure that the electromagnetic spectrum is used more extensively.
Key sensor technology trends include:
(a) Sensors with improved geometric/radiometric performance;
(b) Better exploited electromagnetic spectrum: ultraviolet (UV), far infrared (IR), microwave (MW);
(c) Hyperspectral UV, visible (VIS), near IR (NIR), IR, MW sensors in geostationary Earth orbit (GEO) and elsewhere;
(d) New space sensors with climate-sensitivity detection capabilities;
(e) State-of-the-art sensors/missions to establish traceability in orbit;
(f) Lidar;
(g) Combination of active/passive techniques;
(h) Expanded polarimetric measurement capability (including SAR imagery);
(i) Polarization or incidence-angle pairing;
(j) Diverse radio occultation techniques;
(k) Near-IR measurements of molecular oxygen and water vapour to provide clear-sky surface pressure and cloud top height estimates with accuracies near 1 hectopascal (hPa) and column water vapour estimates with accuracies near 1 millimetre.
CGMS Baseline
The CGMS Baseline enumerates the observations, measurements, and services that form the CGMS contribution to the space-based Global Observing System and is responding to end-user requirements expressed in WMO’s Rolling Review of Requirements (RRR). The CGMS Baseline will strive to support the WMO Integrated Global Observing System (WIGOS) 2040 vision and serves as CGMS’s response to the WIGOS 2040 Vision to document what missions are currently being, or planned on being flown.
The CGMS Baseline included missions that are comprised of the following key principles:
- Commitment: The CGMS Members are providing, or have firm plans to provide, the observations, measurements, and services enumerated in the Baseline.
- Sustained: The observations, measurements, and services are provided on a sustained basis.
- Available: The observations, measurements, and services are available on a free and unrestricted basis.
- Operational: The data and products can be utilised in operational applications.
The observations and measurements that constitute the CGMS Baseline are enumerated in a table that describes the sensor type, the orbit, the observation/measurement (or geophysical parameter), and any specific orbital attributes. The table was designed to facilitate the linkage with the WMO OSCAR/Space database, future risk assessments, and gap analyses.
The CGMS Baseline discusses the importance of services and steps CGMS will take to ensure the quality and continuity of data and products.