WMO ABO Newsletter - Volume 26, December 2024
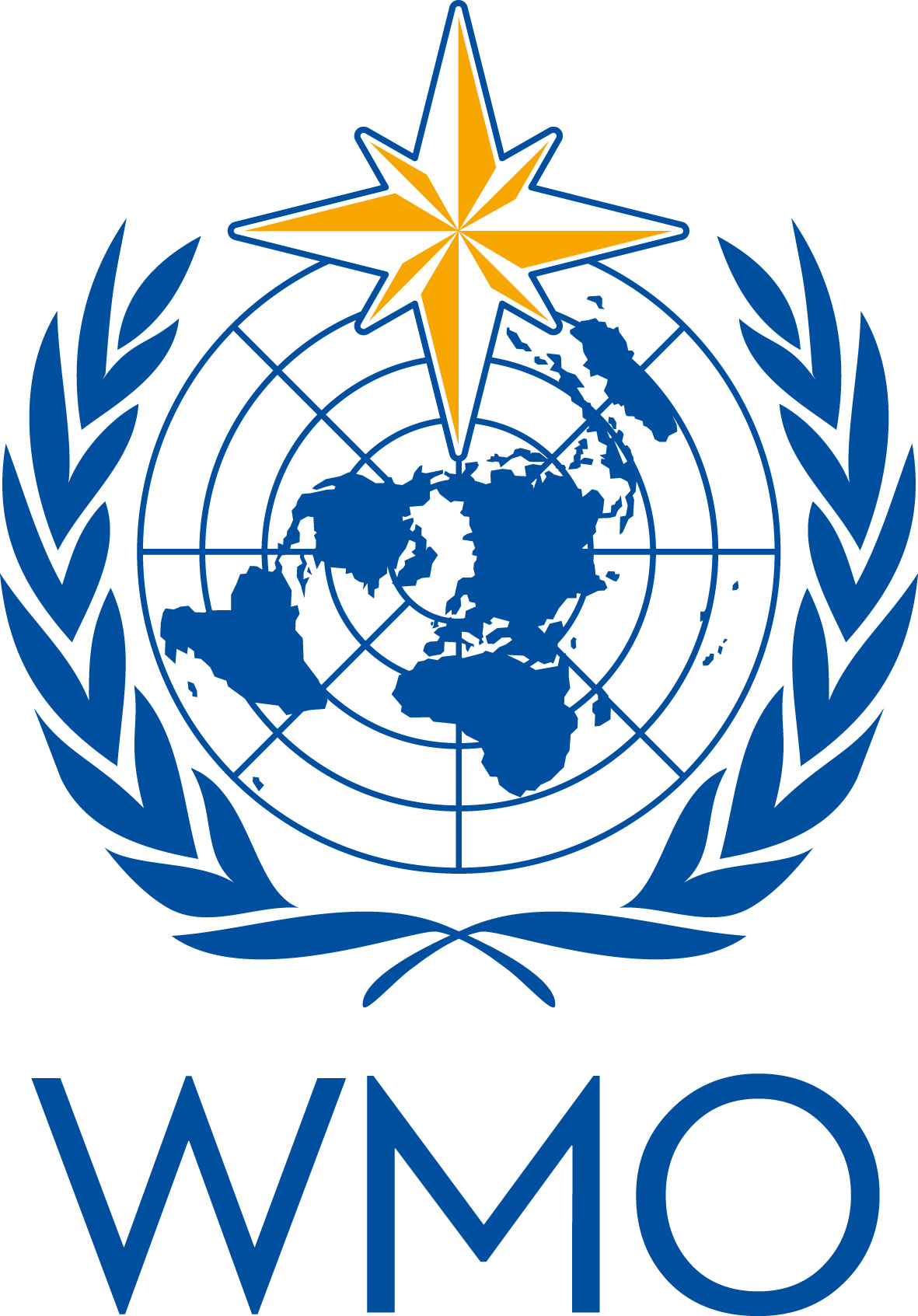
WMO Aircraft-Based Observations Newsletter
Volume 26, December 2024
Contents
- Welcoming Remarks
- Progress in ABO Development and ABO-MR
- Status of Aircraft-Based Observations
- Regional Programme status and developments
- ABOP Water Vapor Measurement Status
- Aircraft-based Turbulence Observations Status within AMDAR
- WMO ET-ABO SG-ADD (Sub-Group - Aircraft Derived Data) Update
- NWP impact: Using Time-Series of Multiple Conventional AMDAR Aircraft Observations to Diagnose the Evolution of Stability and Vertical Motions Prior to Tornado Development
- Operational Example of ABO Data Usage in Forecast Applications: US NWS Storm Prediction Center Uses AMDAR in Severe Weather Forecasts
- Status Report on WMO Uncrewed Aircraft Systems Demonstration Campaign
- Closing Thoughts
- Contacts
- Subscription & Links
Welcoming Remarks
On behalf of the ET-ABO co-vice-chairs and the WMO Secretariat, welcome to the December 2024 edition of the WMO Aircraft-Based Observations (ABO) Newsletter.
As many of you are aware, after 12 years with WMO, Dean Lockett, Scientific Officer, has accepted a position with the Bureau of Meteorology and returned to Australia over a year ago. I am pleased to announce that Mr. Nicolás Rivaben has been selected as Dean’s successor at the WMO Secretariat. In fact, Nicolás has been in the position for many months, but this is our first newsletter published since his selection. Many of you already know Nicolás from his previous role as ABO lead for Argentina’s Servicio Meteorológico Nacional and WMO Region III, as well as serving as Co-Vice-Chair of the ET-ABO. Needless to say, Nicolás has hit the ground running and we are in good hands. A belated welcome, Nicolás!
Along with Nicolás moving into his new role, I also am pleased to announce that Mr. Humphrey Geoffrey Angulu, ABO Lead for the Kenya Meteorology Department and WMO Region I, has agreed to step into a new position as the Co-Vice-Chair alongside Carmen Emmel from Deutscher Wetterdienst. Welcome, Humphrey!
In the last year we have increasingly returned to in-person engagement and meetings. In December 2023, Deutscher Wetterdienst hosted a wide-ranging meeting covering developments in water vapor measurement with emphasis on the emerging topic of contrail monitoring and mitigation and its possible implications for the buildout of additional sensors.
From 10-12 December 2024, the Task Team on Aircraft-based Observations for Region III (TT-ABO III) and the Expert Team on Surface-based Observations and WICAP for Region IV (ET-SBW IV) will host the WMO Regional Workshop on Aircraft-based Observations for the Americas, Panama City, Republic of Panama. We look forward to seeing many of you there virtually or in person. Please stay tuned for more announcements of in-person and virtual events in 2025.
In this edition of the newsletter, we provide updates on regional ABO programmes, water vapor sensing, Aircraft Derived Data, turbulence, the Uncrewed Aircraft Systems Demonstration Campaign (UAS DC) and recent forecasting applications utilizing ABO. We thank the authors for their time and effort in contributing to this newsletter. We further thank Carl Weiss for his effort over the past decade plus in compiling and editing this Newsletter
Curtis Marshall, Chair, ET-ABO
Progress in ABO Development and ABO-MR
ABO-MR
Aircraft-based Observations Metadata Repository (ABO-MR) development -started in 2022- was finally launched as pre-operational mode after ET-ABO I virtual meeting. Several discussions with ABO Managers were addresses such as API issues and minimal metadata list required for ABO Programmes. All ABO Managers are required to join and upload minimal metadata required into the ABO-MR according Guide provided. This metadata will be used by NWP Centres to track any issues related to ABO data sources. Please contact Secretariat for any concern related to ABO-MR.
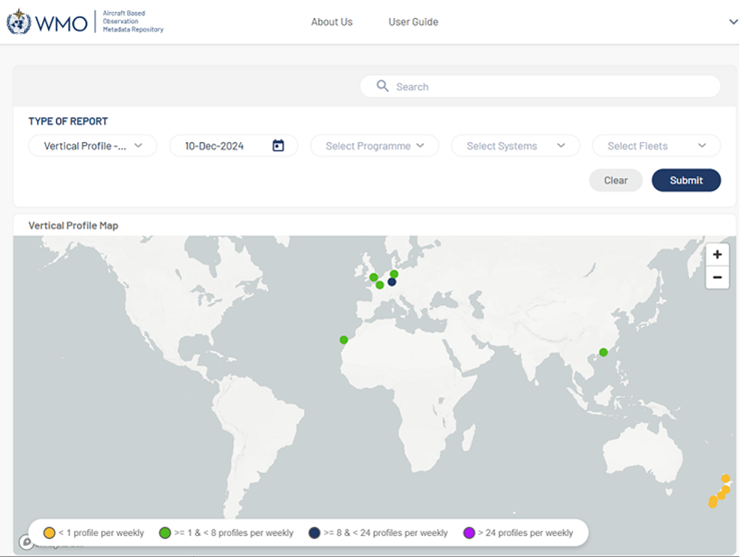
Figure 2-1. Portal of Reports - ABOMR | WMO
WMO-IATA Agreement
As you may be aware, WMO-IATA Collaborative Programme agreement expired last 26 of October with no renovation. It was proposed a new Memorandum of Understanding (MoU) which is under discussion between both organizations.
Development Programmes
Former JET-ABO (CG-WICAP) decided to support programme of data-sparse areas using AMDAR Trust Fund over Region I and Region III. Following this action, new ET-ABO LG decided to support installation of AMDAR Onboard Software (AOS) over 5 aircrafts of Kenya Airways over Africa. Also, it is expected to support cost related to communication cost for selected South American airlines in the near future. WMO is committed to supporting the extension of the E-ABO programme to Regions I and III for the intersessional period 2024-2027.
Nicolás Rivaben, WMO Secretariat
Status of Aircraft-Based Observations
The graphic below shows the smoothed monthly average of daily aircraft-based observations (single point measurements in space and time, mainly of air temperature and wind speed and direction, made by an aircraft) transmitted on the World Meteorological Organization (WMO) Global Telecommunications System (GTS) since 2007 up to October 2024, as contributed by:
All aircraft and all systems (black);
From the AMDAR Programme with reports submitted in binary format (BUFR, red);
From ICAO data sources (AIREP and ADS, purple); and,
From the FLYHT AFIRS system (green).
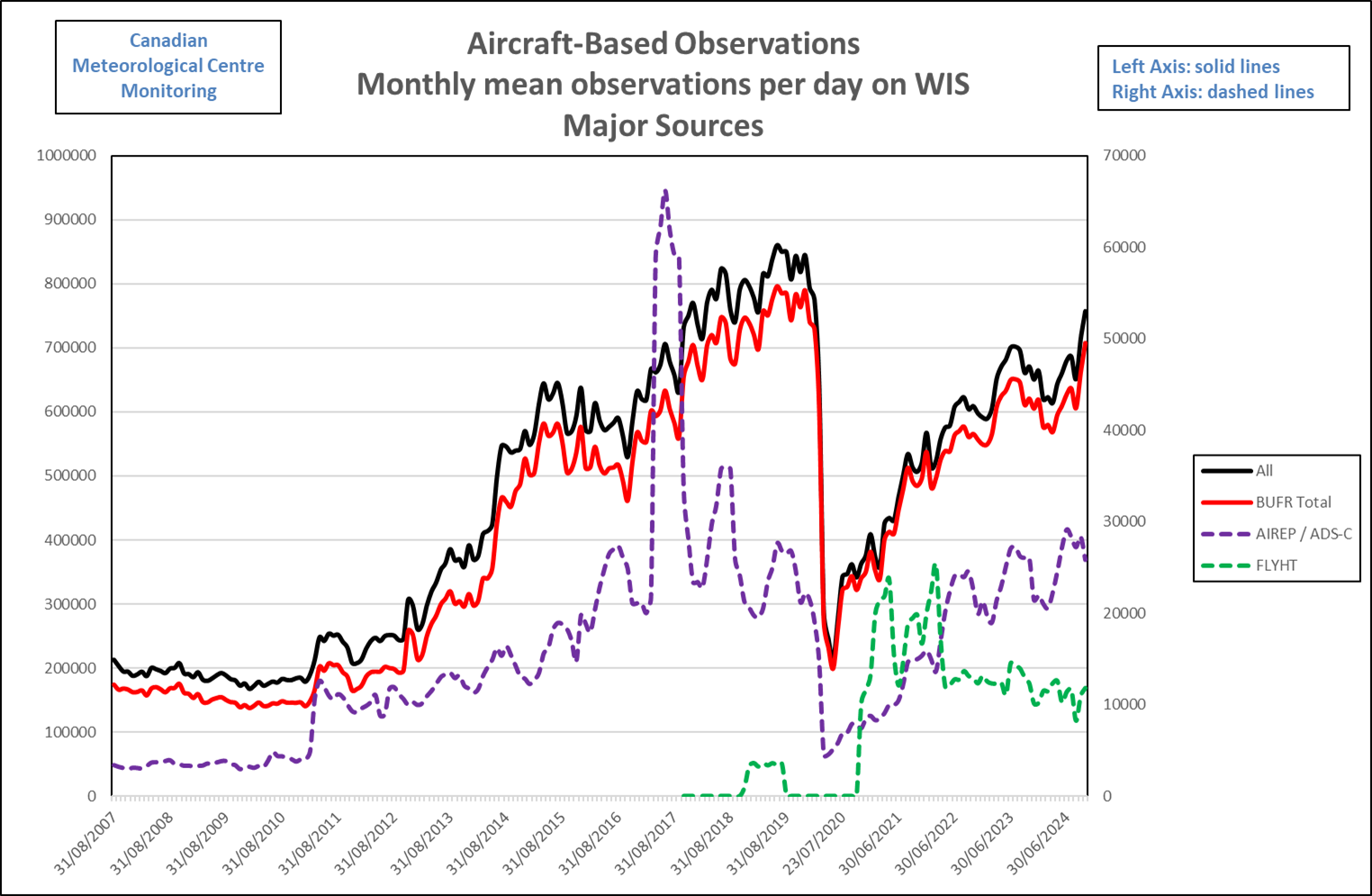
Figure 3-1. Evolution of major sources of ABO from August 2007 to October 2024. Source: CMC data.
The observations from the WMO AMDAR observing system are the output from 12 operational AMDAR national and regional programmes in cooperation with some 43 national and international participating airlines, as listed on the WMO website.
ABO Data Volumes and Coverage
The graph shows that recently, towards the end of 2019 and the beginning of 2020, the volume of data from aircraft-based observations had increased to a historical maximum of more than 850k observations per day on the GTS, with AMDAR observations accounting for the majority of these at around 750k observations per day.
From March 2020, as a direct result of the impact of COVID-19 on the aviation industry and airlines, the volume of aircraft-based observations fell to around 245k observations per day overall and to 230k for AMDAR - around 30% of the December data volume for both.
Since then, data volumes have gradually increased overall, with the average daily total reaching around 750k observations per day in October 2024, which is around 88% of the December 2019 data volume. However, some programs have not yet resumed in areas with sparse data, and it's expected that they will resume next year,
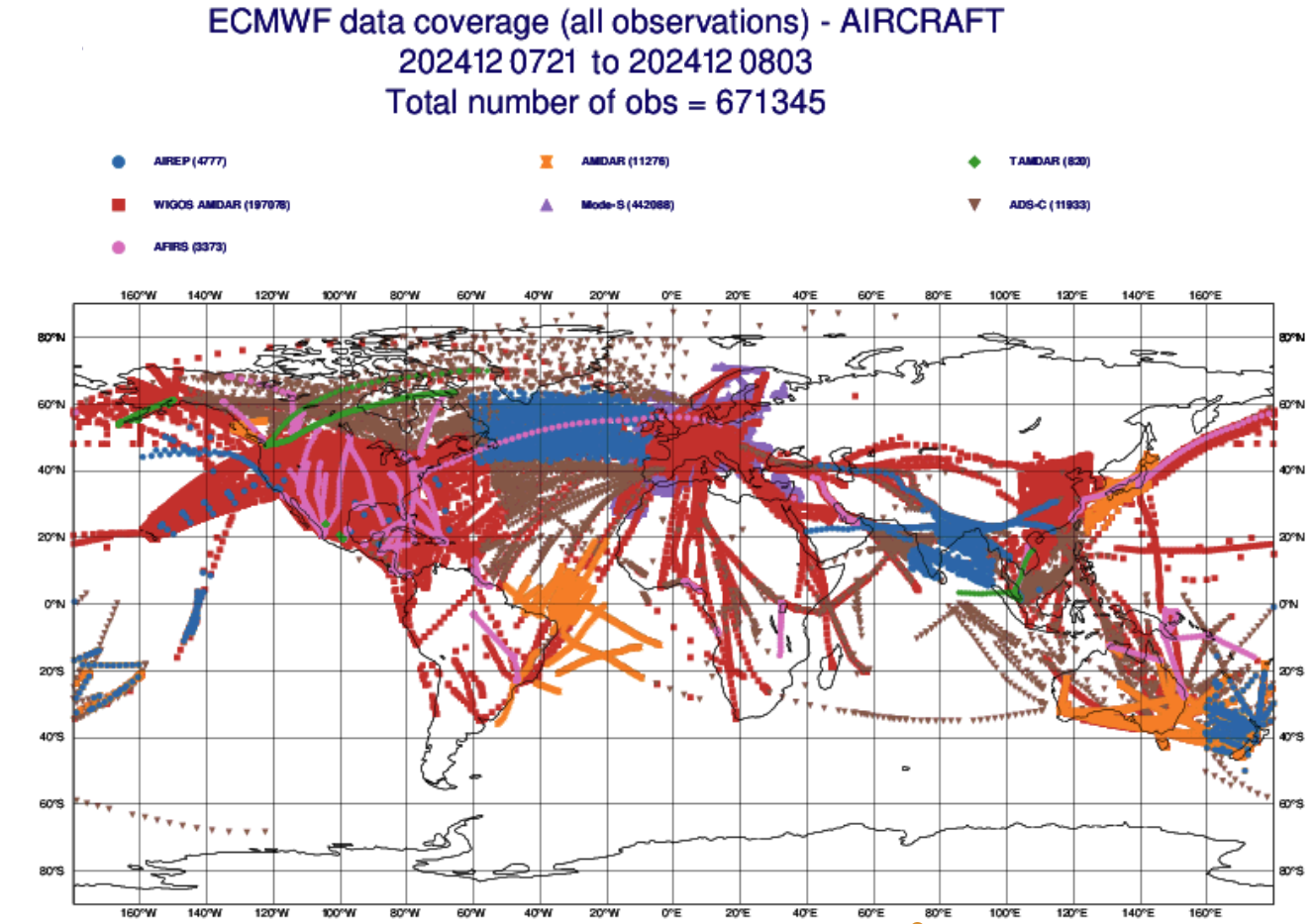
Figure 3-2. Coverage ABO for 6hr. Source: ECMWF
The graphic above, courtesy of ECMWF, provides an indicative coverage of the full ABO observing system over a 06-hour period, including AFIRS, Mode-S, TAMDAR, ADS-C, AIREP and AMDAR (FM42) and WIGOS AMDAR (FM94 BUFR)
The graphic below shows ABO data volumes by WMO region since January 2018, where it can be seen that the recovery varies greatly from region to region and on a monthly basis within each region, as countries and regions emerge from the crisis and aviation operations start to increase accordingly.
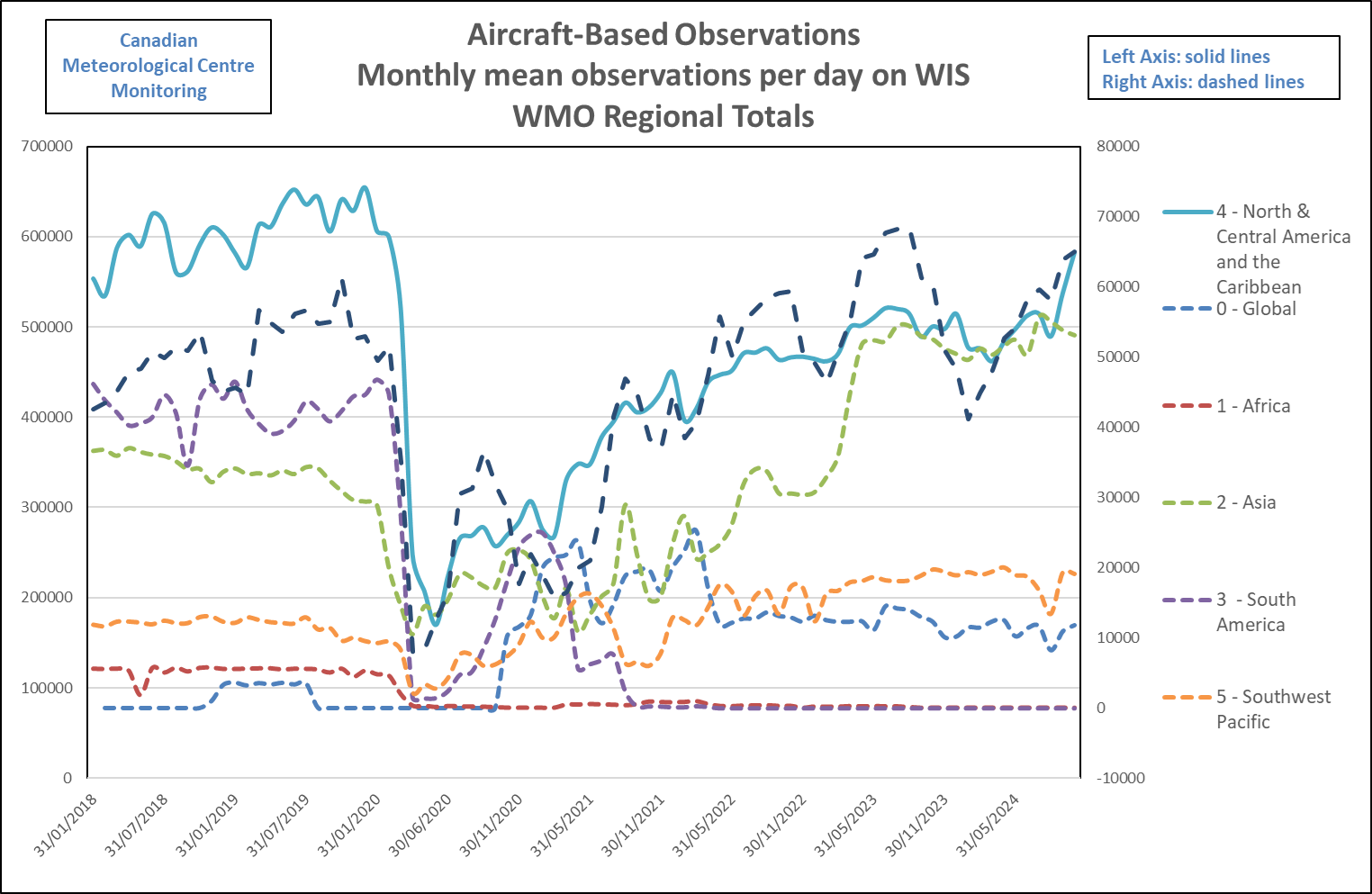
Figure 3-3. Evolution of ABO data from WMO Region between January 2018 to October 2024.
The greatest impacts have occurred in the southern hemisphere where programme and data loss over Africa and South America has been significant. Fortunately coverage has been to a limited extent offset by several measures including:
Ongoing provision of EUMETNET/E-AMDAR data over Africa as a result of WMO AMDAR Operating Fund support;
Installation of AMDAR Onboard software over 5 aircrafts of KQ Airlines -supported by WMO AMDAR Operating Fund support;
E-AMDAR extend coverage over Norther Africa and South America.
USA support for provision of global FLYHT TAMDAR and AFIRS ABO;
Thanks to Program Partners
WMO and its Members are grateful to our aviation industry and airline partners for their continued contribution to the WMO Aircraft-based Observing System and the AMDAR Programme. The data that are produced from this collaboration are utilized within many meteorological applications and forecasts, benefiting aviation operations and safety, other application areas and the wider general public.
For more information on aircraft-based observations data statistics visit the WMO Community Platform Aircraft-Based Observations site.
Regional Programme status and developments
Region I
Kenya
The Kenya AMDAR Programme, with the support of WMO, is in the process of adding five additional B737 aircraft to its fleet and it is envisaged that by June 2025, the programme will have seven aircraft providing data to the WMO Information System (WIS). The Kenya AMDAR Data Processing System (KADPS) is on trial mode and is able to transmit K-AMDAR data to the GTS. Currently, the programme produces an average of 1900 reports weekly and around 10 profiles per week at the Nairobi airport alone. WMO still is supporting the ongoing communications costs for the two B737 reporting aircraft. Plans are being made to expand the fleet to include equipping the rest of the KQ B737 and Embraer 190 aircraft. This is subject to requisite funding becoming available to support both the implementation and communications costs.
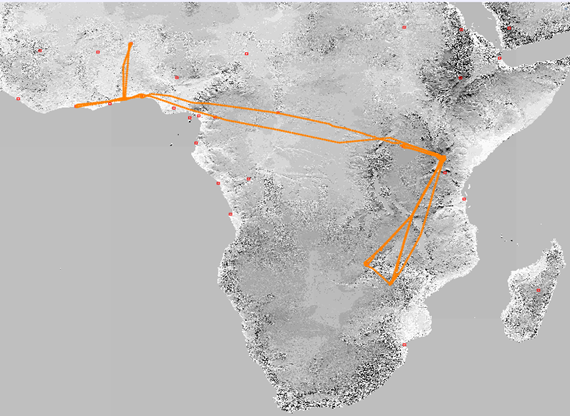
Figure R1. Two weeks coverage of the K-AMDAR Programme (November 1-14, 2024)
Ethiopia
Following the meeting of the RA-I Task Team on Aircraft-Based Observations (TT-ABO) and a workshop on WICAP held in Addis Ababa, Ethiopia in December 2022, more discussion is needed between the parties and the WMO Regional Office for Africa to establish a way forward to implement the programme. Ethiopian Airlines coverage across RA-I is vast and having its participation will greatly improve upper air data for the region.
Morocco
Plans to have EUMETNET, Royal Air Maroc (RAM) and Météo Maroc partner to integrate RAM into the EUMETNET E-AMDAR Programme based on equipping RAM's B738 fleet have not progressed significantly due to changes in key personnel both at EUMETNET and Météo Maroc. It is hoped that with the personnel now in place, this process will move forward.
Egypt
Egypt Air is one carrier that was rated as a high-priority by the analysis of target airlines in WMO RA-I. The Egypt Meteorological Agency and Egypt Air discussed joining the RA-I AMDAR Programme. Egypt Air was receptive to this proposal but expected the Egypt Meteorological Agency to fund the AOS installation. This was not possible at the time due to budgetary constraints.
Humphrey Angulu, Co-Vice-Chair ET-ABO, ABO Focal Point Kenya Meteorological Department (anguluhg@gmail.com)
Region II
From 27 to 30 November 2023, a WIGOS Implementation Workshop for WMO RA-II (Asia) was held in Beijing. Meteorologists and scholars from 22 countries and territories participated in the event. Discussions during the workshop focused on the WICAP regulatory framework, progress on WICAP implementation in RA-II and the aircraft-based water vapour measurement (WVM) system for aviation applications. Difficulties encountered during WICAP implementation were identified and summarised as recommendations for further consideration. You can check at:
https://wmo.int/media/news-from-members/wigos-implementation-workshop-wmo-ra-ii-held
The RA-II Operating Plan (2024-2027), in terms of infrastructure, was discussed and updated in July 2024. RA-II ET-WICAP will continue to develop and promote the programme in the region while supporting AMDAR-related workshops and training.
Yiu-fai Lee, Hong Kong Observatory/Region II ET-WICAP Leader (yflee@hko.gov.hk)
Region III
Last year, Task Team ABO RA-III membership underwent some changes due to personnel reassignments. The new TT members will need some time to adapt to their new roles.
Air operations in RA-III have shown signs of recovery. However, the AMDAR program has not been reactivated by the airlines because of uncertainty in continuing in the ABO program. A collaborative effort is being planned between Regions III and IV to promote the involvement of airlines in the AMDAR regional program particularly in data sparse areas.
The current national status of the regional members is presented below:
Argentina
There is no progress in AMDAR onboard software installations in the B737MAX and E190 fleets due to internal company policy changes.
Furthermore, the Servicio Meteorológico Nacional (SMN) is processing real time data from E-ABO aircraft which fly over Argentine airspace.
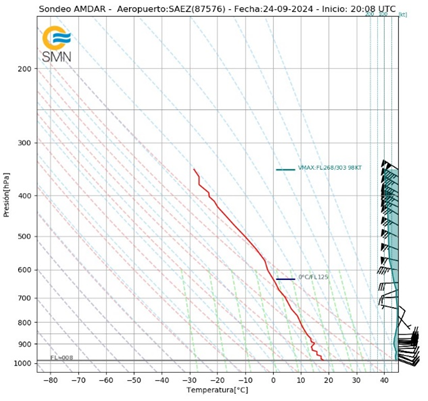
Figure R3-1: AMDAR sounding from the E-ABO programme processed by SMN Argentina.
Brazil
The Brazilian ABO program collects aircraft meteorological observations using two different methods of data linkage: ADS-C and AMDAR. The data gathered by ADS-C through FIR-Atlântico (FIR-AO) show increases every year. Currently, data volume averages approximately fifty thousand messages monthly, occasionally reaching nearly eighty thousand messages per month.
The table below shows the monthly AIREP-UU at FIR-AO messages collected in 2024 by ADS-C.
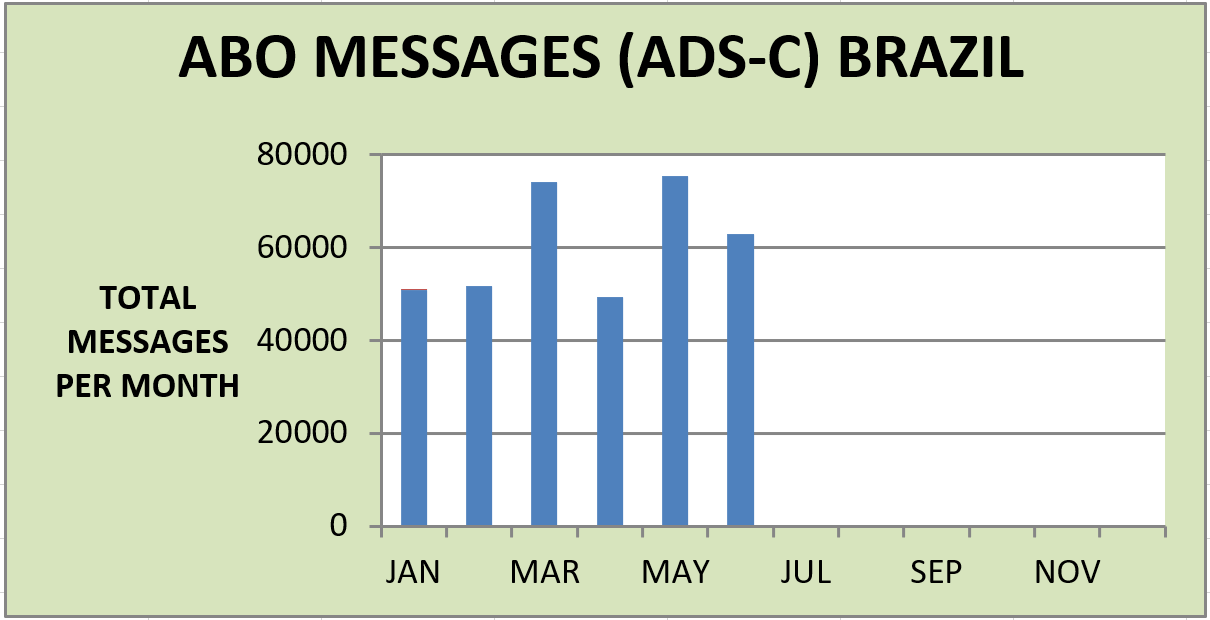
Figure R3-2: 2024 ADS-C Messages from the Brazilian ABO Programme.
The Brazilian AMDAR project is supported by AIRCOM SERVER IN CLOUD service of SITA. The data have been collected by LATAM airlines. However, since the end of 2021 due to the consequences of the COVID-19 pandemic, data provision has stopped resulting in no AMDAR reports being collected in 2024.
The Airspace Control Department (DECEA) of the Brazilian air force has been negotiating an agreement with national carriers AZUL, GOL and LATAM-BR to collect AMDAR data by means of the airlines’ aircraft. However, the main hurdle always has been the data downlink costs. It is worth mentioning that DECEA also is arranging for the inclusion of Brazilian air force aircraft data into the AMDAR program.
Chile
For the last year, the AMDAR program has been stagnant due to the airline´s focus on cost reduction.
Colombia
Collins Airspace and Avianca currently are involved in conversations intended to launch the airline’s participation in the AMDAR program. These arrangements also are expected to lead to aircraft software updates over the next months.
Peru
The Servicio Nacional de Meteorología e Hidrología (SENAMHI), currently is working to launch its “Unmanned Aircraft System” (UAS) which includes a “Meteodrone” from Meteomatics. This system is designed to measure meteorological parameters such as wind speed and direction, temperature, humidity, and atmospheric pressure from the surface to an altitude of 6000 meters.
Hugo Rosado Soto, Chair, Expert Team ABO WMO Region III (hrosado@mtc.gob.pe)
Region IV
The RA-IV Program consists of the USA and USA-based AMDAR enabled airlines, FLYHT TAMDAR and AFIRS-AMDAR, Mexico and AeroMexico as well as Canada and Canadian data sources from FLYHT. The USA AMDAR program additionally provides ADS-C en route wind and temperature reports roughly every 14 minutes on transoceanic and long-haul routes around the globe.
As of November 2024, the USA continues to support the WMO Secretariat and RA-I members to potentially obtain and provide AMDAR from Kenya Airways. The WMO Secretariat will fund additional avionics capabilities for the Kenya Airways fleet and when the data become available, the USA will provide them as part of its USA ABO feed to the Global Telecommunications System/second generation WMO Information System (GTS/WIS 2.0).
The USA ABO and National Mesonet Programs secured funding through April 2025 to continue providing TAMDAR and AFIRS-AMDAR from FLYHT. Please see the water vapor report in this newsletter for an update on FLYHT TAMDAR. Additionally, the USA is working with FLHYT to fund the installation of an additional five WVSS sensors on WestJet by the end of 2025 with a total of 10 new installations by that time. These aircraft will service the USA west coast and routes to the central tropical Pacific Ocean.
The USA continues its partnership with the Massachusetts Institute of Technology Lincoln Labs to prototype the Portable Aircraft Derived Weather Observation System (PADWOS) for obtaining Mode-S wind and temperature observations at select locations in the USA. Please see the special feature article on Aircraft Derived Data in this newsletter edition for additional information.
Canada is working actively to expand its AMDAR capability through partnerships with data providers and airlines. Unfortunately, on June 30, Jazz Aviation LP withdrew the last AMDAR-reporting CRJ-200 from its fleet ending 20 years of CRJ-200s reporting under the Canadian program. I would like to thank Jazz and their CRJ-200 fleet for the long and fruitful relationship with Environment and Climate Change Canada (ECCC) over the last two decades which allowed Canada to join the AMDAR data provider community. Again, Thank You!
As a mitigation to the above news and to sustain the Canadian program, ECCC is working on a continuity plan targeting the capacity of Jazz’s CRJ-900 fleet in order to maintain the supply of ABO data across Canada. Discussions between ECCC, Jazz and Rockwell Collins have been ongoing since the summer of 2024. The expansion objective still is to give priority to the northern latitudes which are recognized as a poor area for ABO and upper air data. Discussions with FLYHT are moving in this direction while new data are being tested by ECCC’s NWP. Further details will be announced at a later date.
Curtis Marshall, USA AMDAR Program Lead (curtis.marshall@noaa.gov)
Frédéric Lenormand, Canadian AMDAR Program Manager
Region V
The number of RA-V aircraft-based observations remains consistent at approximately 35k reports per day from
RA-V NMHS programmes [New Zealand, Australia]
FLYHT/AFIRS, and
"Visiting" AMDAR equipped Aircraft.
The RA-V Expert Team on the WMO/IATA Collaborative AMDAR Programme (ET:WICAP) will meet in Q3 2024. Currently, the team is considering:
what benefits their NMHS could derive from a regional aircraft observations programme, and
what contribution their NMHS could make to such a regional programme.
Dr. Doug Body, Bureau of Meteorology (douglas.body@bom.gov.au)
Region VI
The EUMETNET ABO (E-ABO) programme manages aircraft-based observations for Europe with a focus on the EUMETNET Composite Observing System (EUCOS) domain (Figure 1). During this programme phase, which runs from 2024 to 2028, the E-ABO programme is being managed by the Met Office in partnership with the Royal Netherlands Meteorological Institute (KNMI) and the German Meteorological Service (DWD). KNMI, through its European Meteorological Derived Data Centre (EMADDC) is responsible for the operation and development of derived ABO from ADS-B/Mode S data over Europe. EMADDC personnel were delighted to win the European Meteorological Society (EMS) Technology Achievement Award 2024.
‘The EMADDC team has demonstrated that an opportunistic use of aircraft data can be turned into a well calibrated, rich source of upper air observations, and that, through international collaboration by EUMETNET, these can be provided as a valuable service to the aeronautical and meteorological communities.’ (Quote from the EMS website).
The AMDAR network has seen a steady growth in data availability as flight schedules have increased. An example of the daily coverage of AMDAR data over the EUCOS area is shown in Figure R6-1 below.
An example of the daily coverage of AMDAR data over the EUCOS area is shown in Figure R6-1 below.
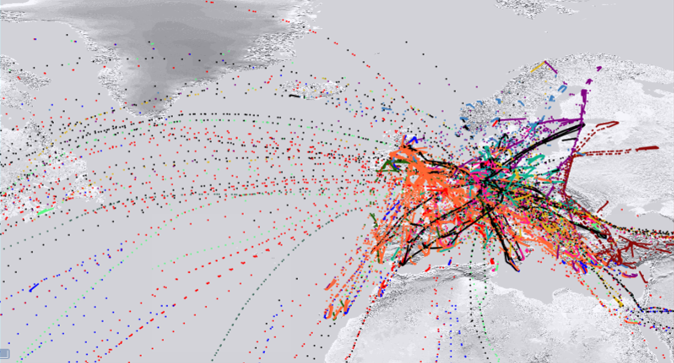
Figure R6-1: AMDAR data coverage over the EUCOS domain for one day.
In September 2024, AMDAR was implemented operationally on the B777 fleet of a new airline, KLM Royal Dutch Airlines. This new data will improve coverage over routes to South America and Africa in particular and help fill the gaps in these data sparse areas. The KLM AMDAR data also includes Global Navigation Satellite System (GNSS) heights which are expected to bring further benefits for NWP (Figure R6-2).
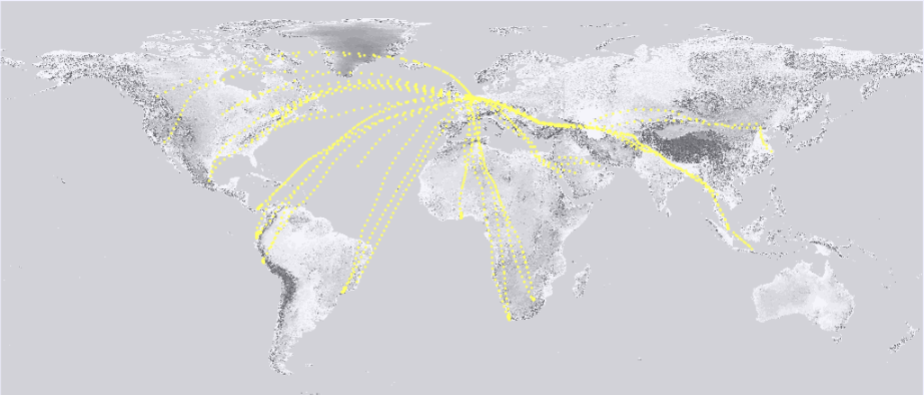
Figure R6-2: Coverage of KLM B777 data for one day.
There have been improvements in the European Mode S derived data coverage through developments in the local receiver networks over the UK, Finland and Norway and the collection of data directly from DSNA (Direction des Services de la Navigation Aérienne) in France. The Met Office and EMADDC recently have implemented derived ABO from global Mode S which extends the coverage significantly and helps fill gaps over what were previously data sparse areas. Refer to the aircraft derived data article in this newsletter for more details.
The European area continues to receive ABO from other sources such as ADS-C, AIREP, AFIRS AMDAR and TAMDAR (Figure R6-3).
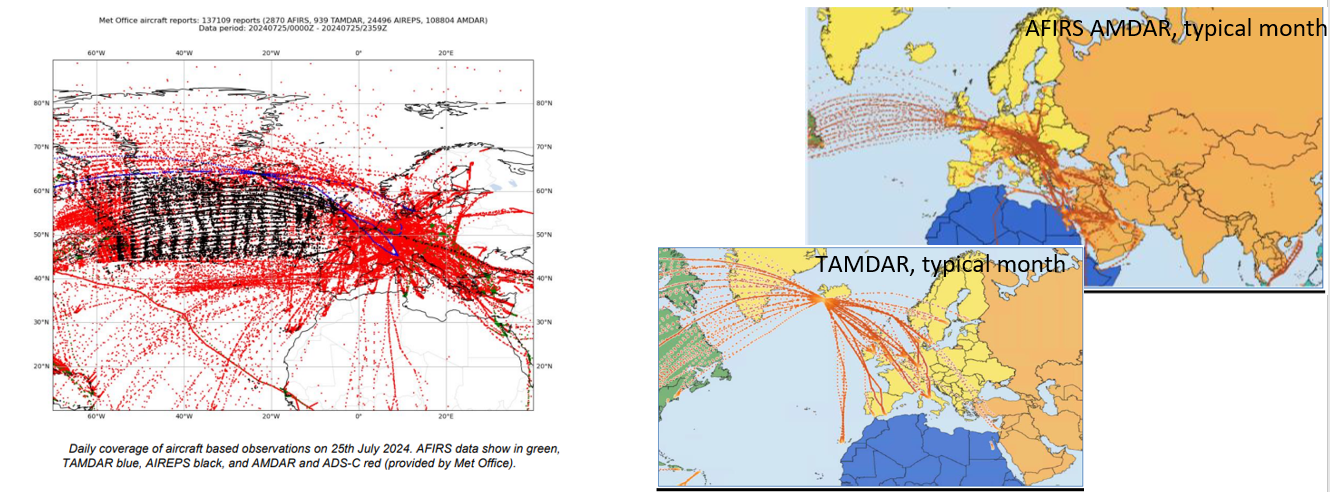
Figure R6-3: Coverage from other ABO data sources in RA-VI.
The Met Office is collaborating with Scottish airline Loganair on a humidity project to install FLHYT WVSS-II sensors on its Embraer 145 fleet. This would deliver a sizeable increase in humidity profiles over the UK which will support improvements in weather forecasting. DWD is working on a contrails project (MEFKON) and is collecting additional en route humidity data at one-minute intervals, available on the WMO Information System (WIS), from the nine Lufthansa aircraft equipped with the WVSS-II sensor. There continues to be significant interest in the climate impacts of contrails and the E-ABO programme manager is following the progress of the SESAR project, CICONIA, through their stakeholder outreach body.
With increases in derived data from Mode S, the E-ABO programme is planning a gradual reduction in AMDAR data over the next few years whilst maintaining a comprehensive ABO coverage. Since September 2023, the time interval for en route data over Europe, which is covered well by Mode S derived data, was increased from seven minutes to fifteen minutes for budgetary reasons.
The total number of observations collected each month is shown in the graph below (Figure R6-4). Note that the scale for Mode S data is in millions!
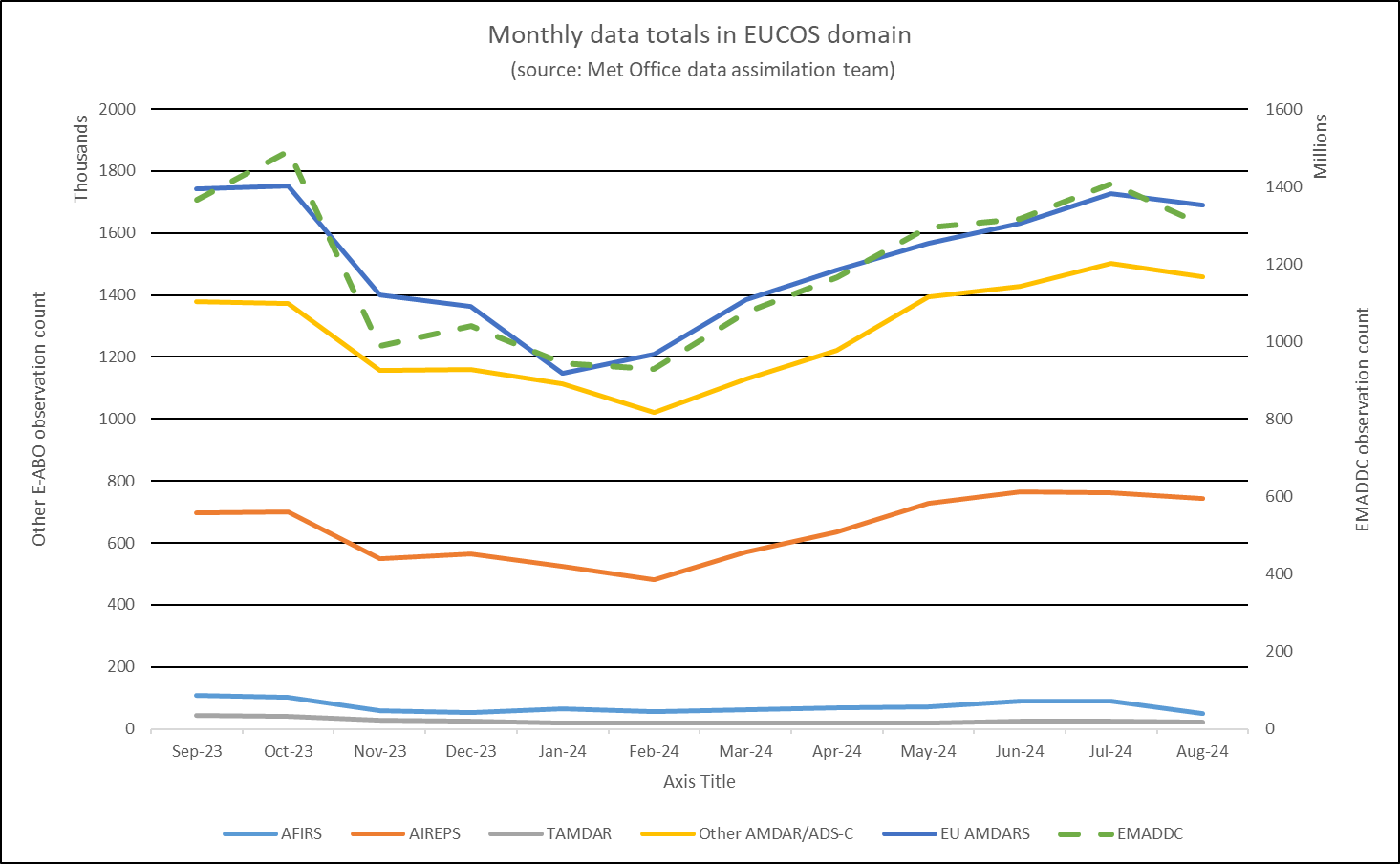
Figure R6-4: Total number of ABO observations each month by data type in the EUCOS domain.
European ABO reports are being affected by GPS spoofing which leads to false locations for the data (see example of AMDAR data below in Table R6-1). The E-ABO team is investigating options for improving the quality control checks to prevent more of these erroneous data from being released to users.

Table R6-1: Example of false location information which is thought to be due to GPS spoofing.
It is common for individual aircraft to move between airlines, or be removed from fleets altogether. So, the E-ABO technical coordinator has undertaken a comprehensive review of the E-ABO programme aircraft list to help ensure the correct allocation of aircraft data to individual airlines.
Finally, the E-ABO programme manager has joined the RTCA/EUROCAE Joint Working Group 76 sub-groups on turbulence reporting and ABO. These groups aim to create a technical standard for turbulence reports and recommendations for more general criteria development for ABO.
Martyn Sunter, E-ABO programme manager, Met Office (martyn.sunter@metoffice.gov.uk)
David Snook, E-ABO technical co-ordinator, Met Office
ABOP Water Vapor Measurement Status
Water vapor measurements have been a part of AMDAR programs almost since the beginning. While for many years the number and distribution of water vapor-equipped aircraft has not changed, new developments are in the pipeline. Currently, 132 Southwest and UPS aircraft in North America and nine aircraft in the Lufthansa fleet in Europe are equipped with the WVSS-II sensor (see table below). The UK Met Office is conducting a project to install 12 new FLYHT WVSS-II on Embraer aircraft belonging to Logan Air with the first installation expected later this year or early in 2025.
Additionally, NOAA plans to outfit ten aircraft with the new FLYHT WVSS-II sensors by the end of 2025. The initial five sensors will be installed on WestJet Airline’s Max-8 and 737-800 aircraft during the first quarter of 2025, while installation of the remaining sensors is planned later during 2025.
While these equipped aircraft fly sporadically outside their usual geographic constraints (e.g., northern Africa and Central America), many areas of the world remain without any AMDAR water vapor measurements.

Table 5-1: Operational WVSS-II units in service to ABOP, by WMO Region.
New developments in the field of contrail avoidance give hope that more installations will be realized in the future. Persistent contrails, especially at night, can have a substantial warming effect. In order to reduce the environmental impacts caused by the aviation industry, the prediction and avoidance of climate sensitive contrails are being investigated through several national and international projects. However, for these purposes, enroute measurements with a higher accuracy than the WVSS-II sensor can provide most probably will be necessary. Many projects like ARPA-E, CICONIA, D-KULT, MEFKON and Horizon BeCom currently are being conducted to investigate the feasibility and impact of contrail avoidance. Several projects also are looking at new sensors or the use of current WVSS-II system to improve the predictions of ice supersaturated regions where contrails can form.
While NOAA’s WVSS-II programme includes ascent and descent profiles as well as enroute humidity data (three-minute resolution for enroute data) over Europe, only ascent and descent profiles usually are provided. Through the MEFKON project, enroute humidity observations from the nine equipped aircraft over Europe are currently available. These data, with a one-minute temporal resolution, started in Q2 2024 and will continue until approximately the end of 2025. These measurements will be used to test if WVSS-II observations can be used as a quick fix to improve the prediction of contrails in regions with a high probability of their formation.
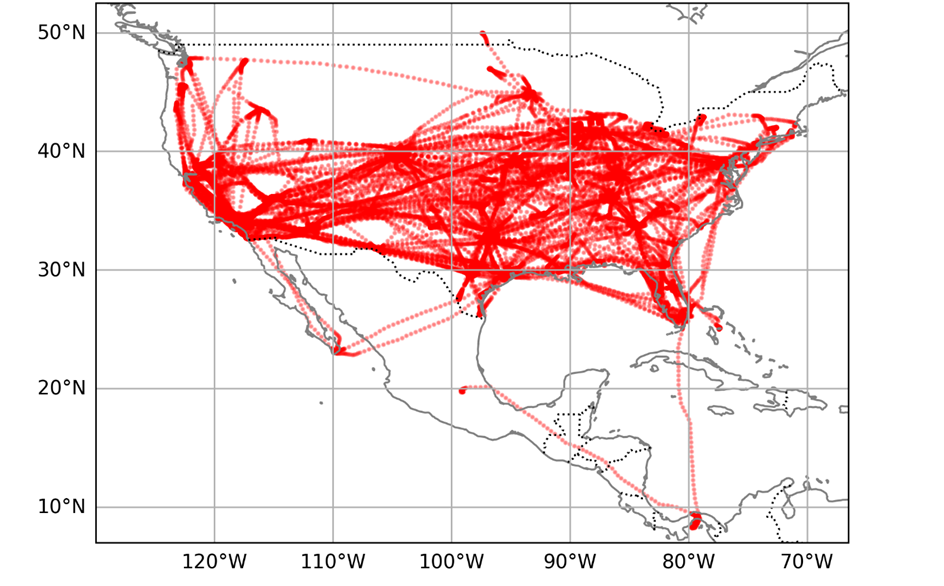
Figure 5-1: WVSS-II measurements over North and Central America on 19 September 2024 (source: NOAA).
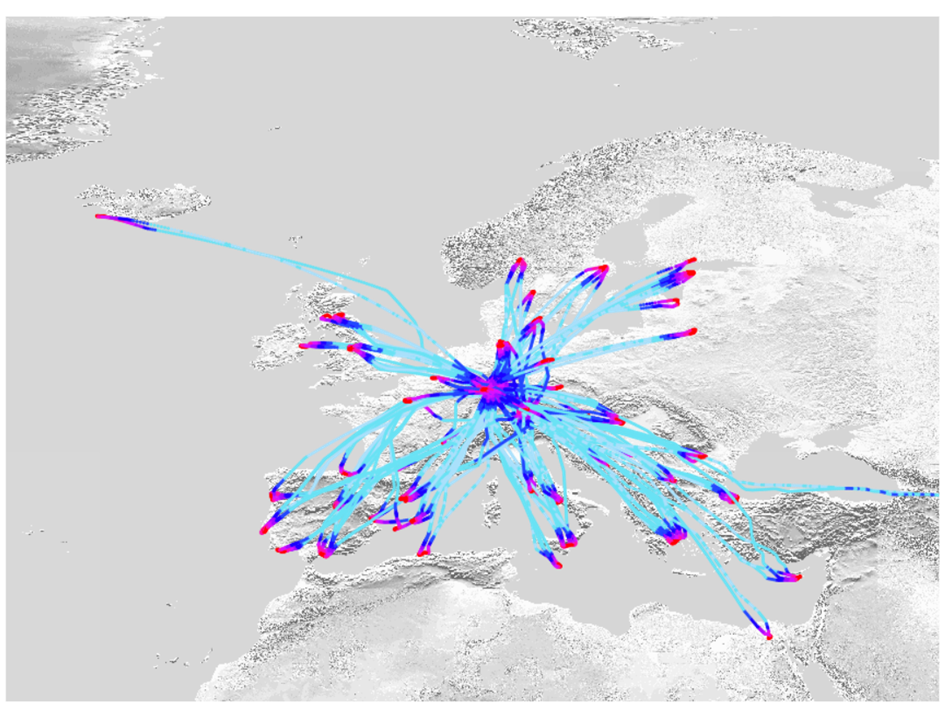
Figure 5-2: WVSS-II measurements over Europe from 25-31 August 2024 (source: E-AMDAR portal, 20.09.2024).
FLYHT TAMDAR Availability
FLYHT currently operates 25 active TAMDAR units. This number has declined notably in recent months due to aging systems and aircraft replacement. The breakdown of installed TAMDAR by WMO region is shown below:

Figure 5-3: Operational TAMDAR Units in Service by WMO Region.
FLYHT’s TAMDAR network produces an average of 110 soundings per day. The map below illustrates the locations of TAMDAR observations for August 2024.
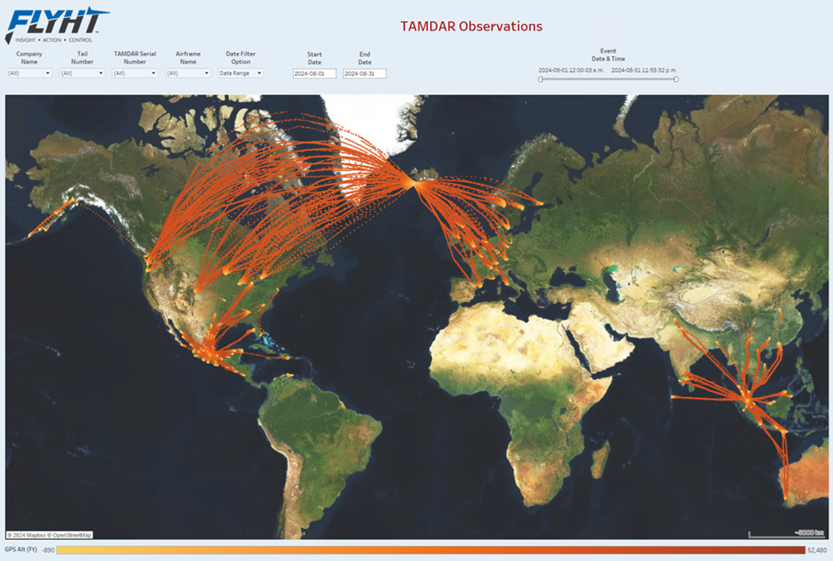
Figure 5-4: FLYHT‘s data coverage during August 2024.
As mentioned, FLYHT will manage two separate sensors, TAMDAR and WVSS-II. FLYHT has no plans to expand the TAMDAR network but is concentrating instead on enhancing water vapor data by expanding the deployment of the WVSS-II sensor.
Carmen Emmel, Deutscher Wetterdienst (DWD), ABO Coordinator (carmen.emmel@dwd.de)
Meredith Bell, FLYHT, Atmospheric Program Manager
Aircraft-based Turbulence Observations Status within AMDAR
There are two atmospheric turbulence intensity metrics currently used by the AMDAR community. The first metric is eddy/energy dissipation rate (EDR). MacCready (1964) first suggested the use of EDR as a measure of turbulence intensity. It is particularly useful operationally since EDR along the vertical direction is proportional to the RMS (root-mean-square) vertical acceleration experienced by an aircraft for any given flight condition (MacCready 1964; Cornman et al. 1995). Further, EDR has been adopted as the standard metric for atmospheric turbulence reporting by the International Civil Aviation Organization (ICAO) (2001).
Figure 6-1 shows the locations of available EDR reports for September 2024. Currently, about 1,900 aircraft are reporting EDR worldwide with an average of over 90,000 observations per day. EDR reporting has increased globally but continues to be low across Africa, Asia and South America.
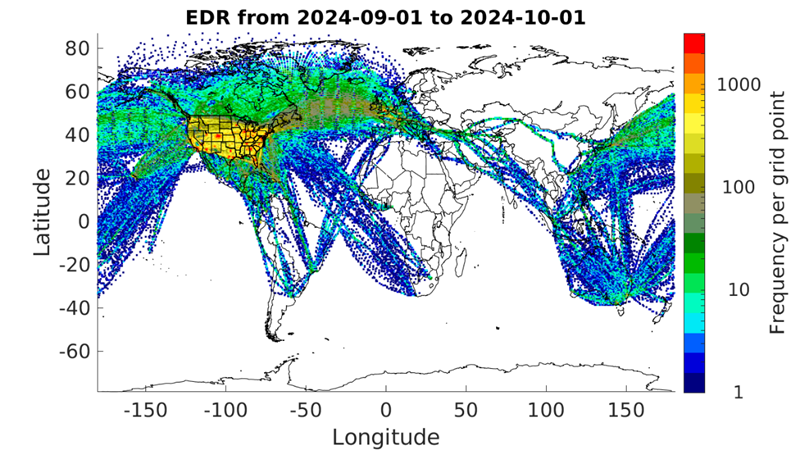
Figure 6-1: Counts of all available EDR reports for September 2024 using an approximately 50x50 km grid. The color scale is logarithmic.
Figure 6-2 shows the number of routine observations per day since May 2023. We show the counts of routine observations, rather than all observations since they are not impacted by the amount of elevated turbulence.
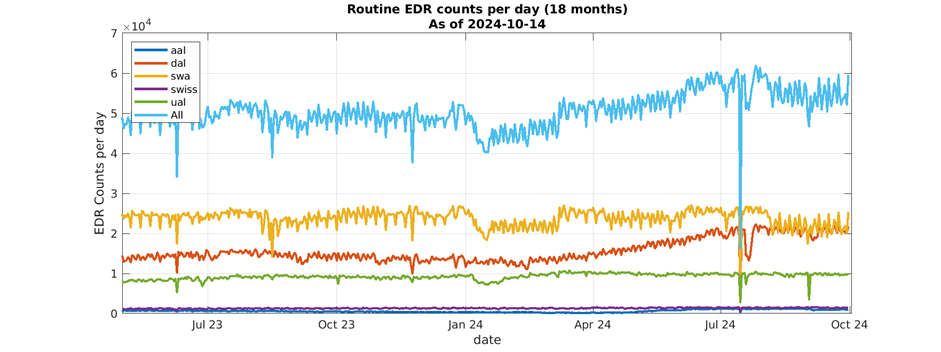
Figure 6-2: Counts of routine daily EDR observations by airline from May 2023 to September 2024.
The second metric, derived equivalent vertical gust (DEVG), is defined as the instantaneous vertical gust velocity which, when superimposed on a steady horizontal wind, would produce the measured acceleration of the aircraft. The effect of a gust on an aircraft depends on its mass, aerodynamic characteristics as well as flight condition, but these factors are accounted for so that a gust velocity can be calculated which is independent of the airframe.[1] Currently, there are at least 150 aircraft reporting about 2,800 DEVG measurements per day. These numbers are based on an analysis of AMDAR data from The U.S. National Centers for Environmental Prediction’s (NCEP) Meteorological Assimilation Data Ingest System (MADIS). The large drop in reporting since the last (April 2023) newsletter appears to be because of the complete absence of reports over Australia. Figure 6-3 shows the locations of all DEVG reports for September 2024. There is software-based logic implemented on many aircraft that limit reporting of DEVG in some regions at certain altitudes (e.g., Europe). DEVG reporting has increased over North America but continues to be limited through Asia and the Southern Hemisphere.
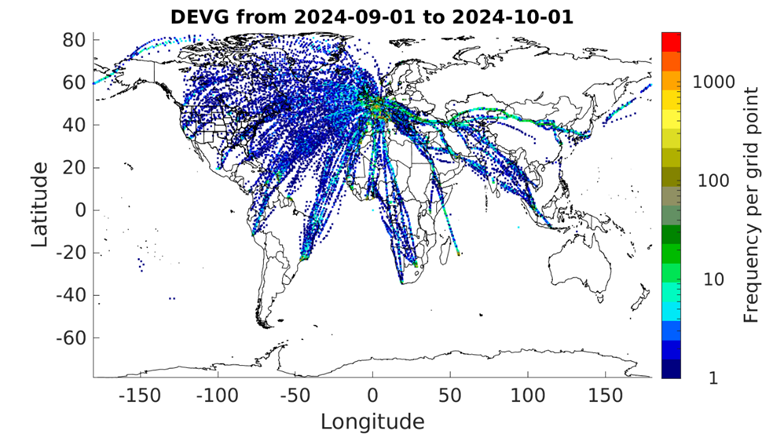
Figure 6-3: Counts of all available DEVG reports for September 2024 using an approximately 50x50 km grid.
These maps illustrate the need for expansion of aircraft-based turbulence observations in many areas of the globe. WMO continues to work with its Members and their respective national airlines to increase aircraft-based turbulence observation coverage.
WMO and its Members express gratitude to our aviation industry and airline partners for their continued contribution to the WMO Aircraft-based Observing System and the AMDAR program. The data produced from this collaboration are utilized within many meteorological applications and forecasts benefiting aviation operations and safety, other application areas and the wider general public.
For more information on aircraft-based observations data statistics visit the WMO website.
Note:
[1] From EDR IP
[2] Not all of the EDR data collected globally are available in this dataset.
[3] From Guide to Aircraft-based Observations, WMO-No. 1200
Gregory Meymaris, National Center for Atmospheric Research (meymaris@ucar.edu)
WMO ET-ABO SG-ADD (Sub-Group - Aircraft Derived Data) Update
There have been a number of aircraft-based observation initiatives this year associated with derived and third-party data. These include improvements to the Mode Selective Enhanced (colloquially Mode S or Mode S EHS) derived data over Europe, the introduction of a global Mode S dataset and the development of PADWOS (Portable Aircraft-Derived Weather Observation System).
The Royal Netherlands Meteorological Institute (KNMI), through its European Meteorological Aircraft Derived Data Centre (EMADDC) is responsible for the operation and development of derived ABO from ADS-B/Mode S data over Europe. The map below (Figure 7-1) provides an example of the derived data coverage over the European area from EMADDC for a single day. There have been developments in the local receiver networks over the UK, Finland and Norway as well as the collection of Mode S EHS data directly from DSNA (Direction des Services de la Navigation Aérienne) in France. The expansion of the UK local receiver network has improved the coverage in the lower troposphere in particular (Figure 7-2) where the data are now available at lower levels in the atmosphere at many airports.
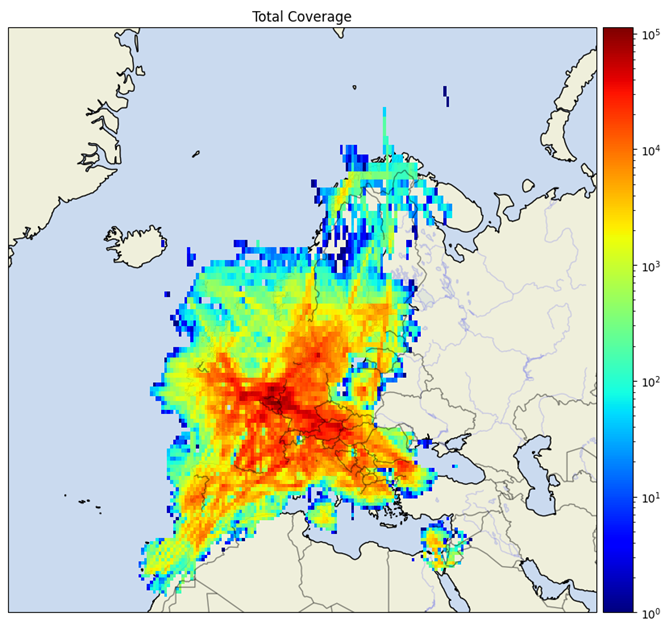
Figure 7-1: Number of derived data observations in 0.5°x0.5°grid box from ADS-B/Mode S for one day (EMADDC European coverage)
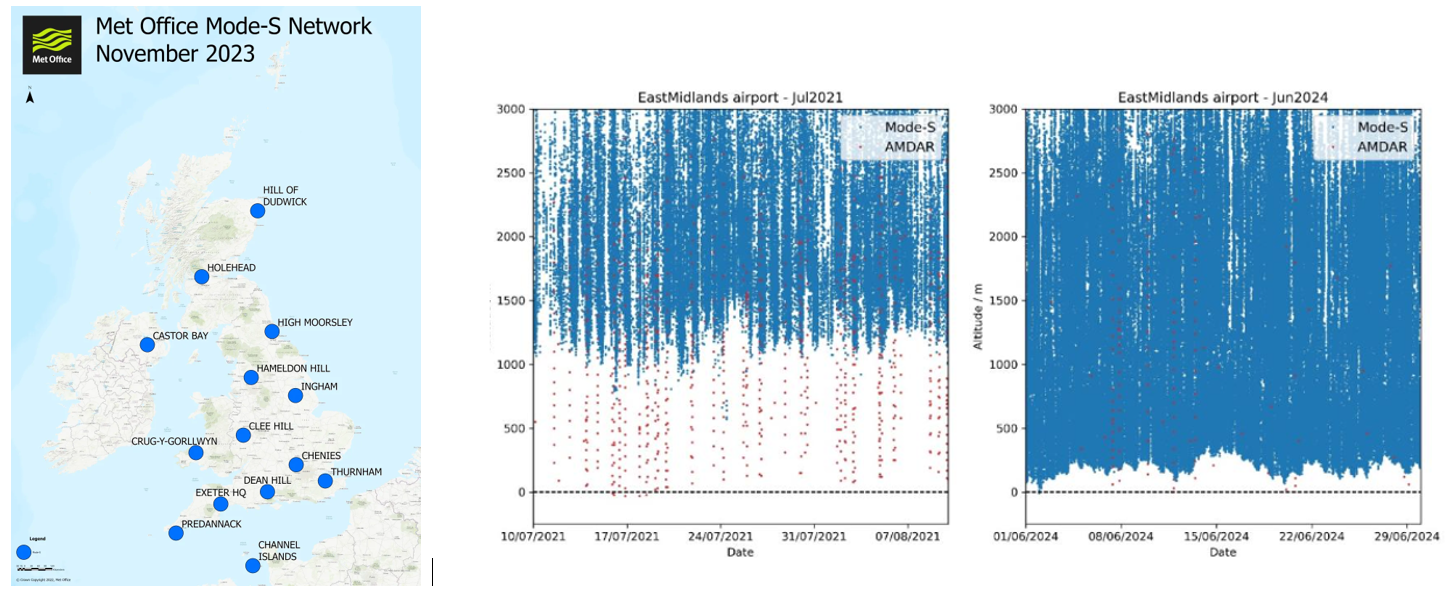
Figure 7-2: UK Mode S network and example of improvement in coverage from East Midlands Airport. The Mode S data now extend to lower levels.
The Met Office and EMADDC recently have implemented derived ABO from global Mode S data which extends the coverage significantly and helps fill gaps over what were data sparse areas previously (Figure 7-3). This is expected to bring further improvements to global and regional NWP. The global Mode S derived data are now available to other national met services. The process to access the data can be found here.
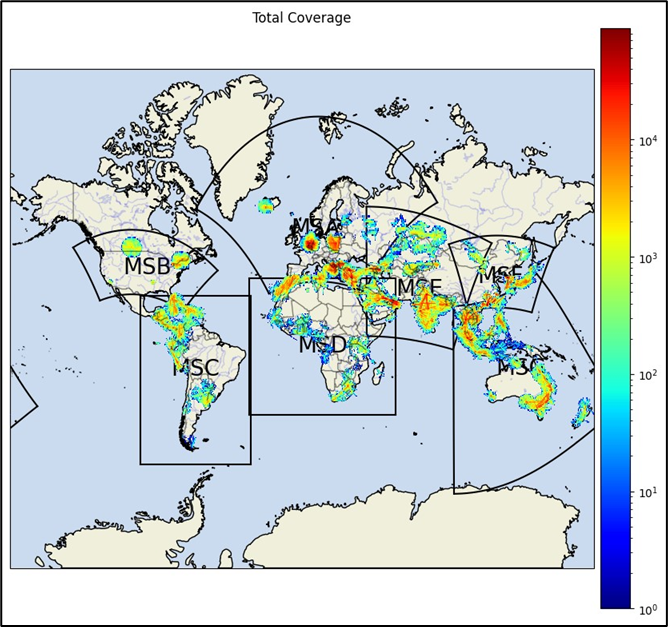
Figure 7-3: Number of derived data observations in 0.5°x0.5°grid box from ADS-B/Mode S for one day (global coverage)
ABO from other sources such as ADS-C, AIREP, AFIRS, AMDAR and TAMDAR continue to be delivered by the Global Telecommunications System/WMO Information System (GTS/WIS - Figure 7-4).
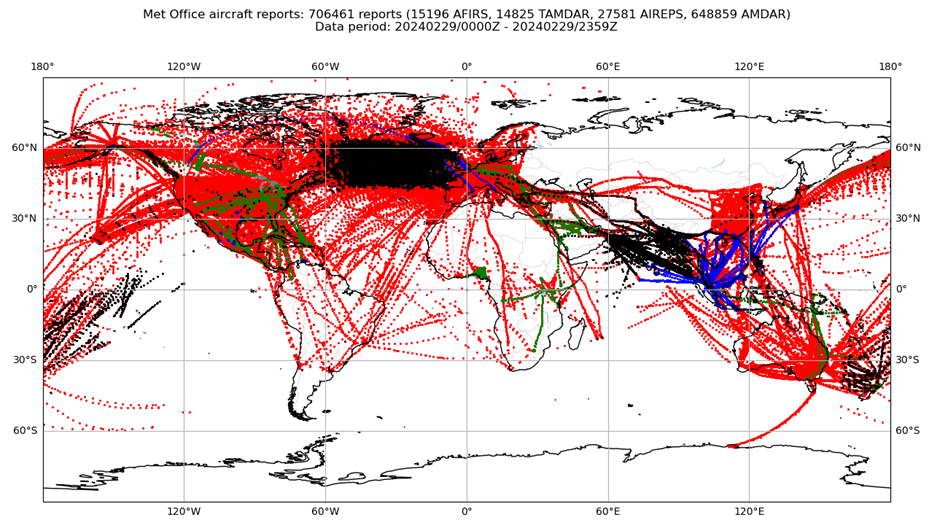
Figure 7-4: Global ABO coverage (excluding Mode S) for a single day. Red: AMDAR and ADS C, Black: AIREP, Green: AFIRS, Blue: TAMDAR
PADWOS (see Figure 7-5 below), developed at the Massachusetts Institute of Technology Lincoln Laboratory, will interrogate aircraft, in a similar way to an airport secondary surveillance radar, to enable the collection of Mode S EHS data. This means high frequency wind and temperature data can be derived from aircraft within the range of the system.
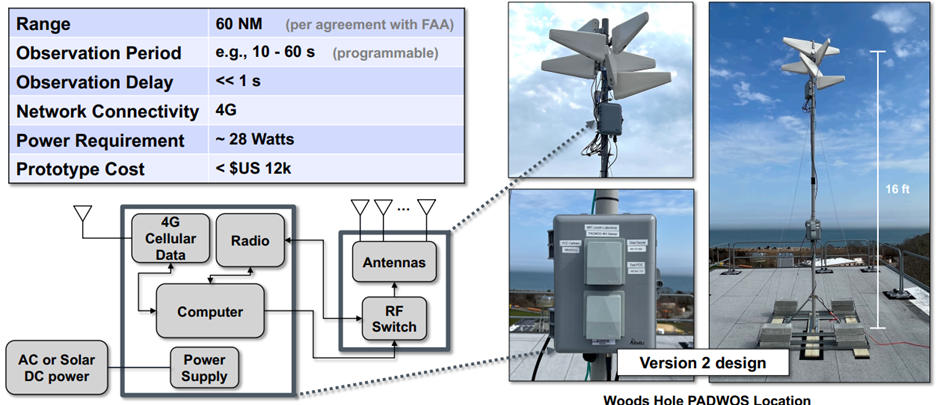
Figure 7-5: Portable Aircraft-Derived Weather Observation System (PADWOS).
Working in partnership with NOAA, six PADWOS sites were established in the USA last year (see Figure 7-6).
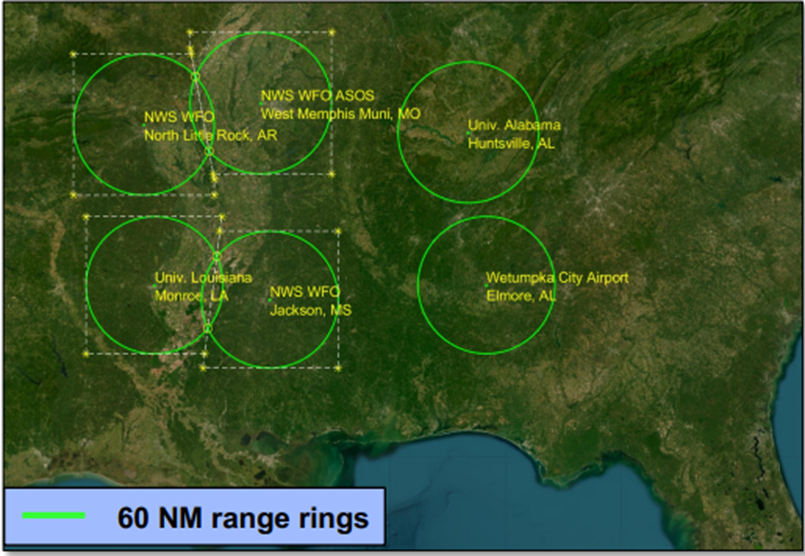
Figure 7-6: PADWOS USA sites
Figure 7-7 gives an indication of the data coverage when compared with the traditional AMDAR data known as MDCRS (Meteorological Data Collection and Reporting System).
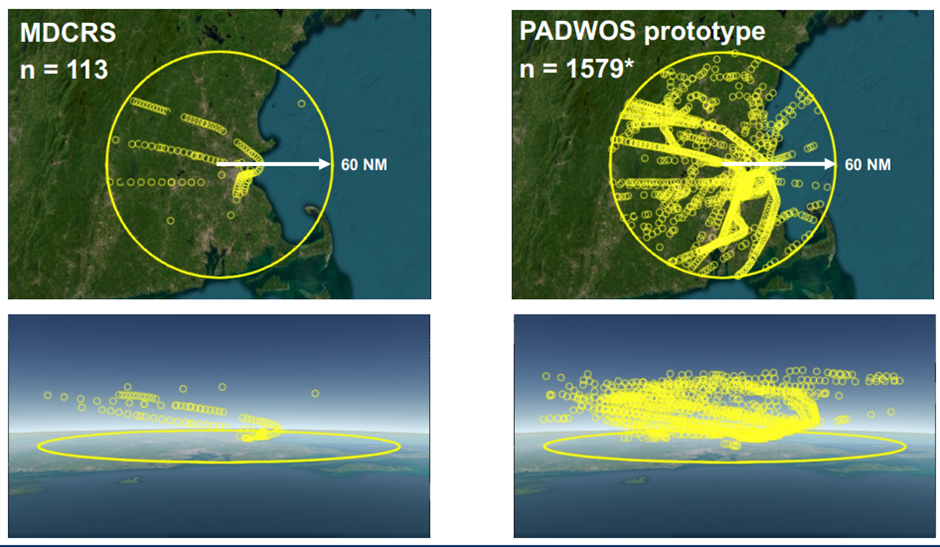
Figure 7-7: Example of data available from 30-minute period comparing traditional AMDAR data (MCDRS) with the PADWOS prototype system.
These systems could be installed in data sparse areas to improve coverage where Mode S data are not collected, though permission would be required first from civil aviation and communications authorities.
Finally, ADS-B version 3 provides the option for aircraft to broadcast weather data in their routine ADS-B messages. Broadcasting a complete set of weather data is an optional capability. However, it is expected that there will be sufficient information within the mandatory elements of the ADS-B messages to derive wind information in the same way that is done currently with ADS-B/Mode S EHS data. It is understood that adoption of the new format requires new hardware on the aircraft and that this is not expected to be available widely for many years.
Martyn Sunter, E-ABO Programme Manager (martyn.sunter@metoffice.gov.uk) - with thanks to Michael McPartland of Massachusetts Institute of Technology Lincoln Laboratory for the information on PADWOS.
NWP impact: Using Time-Series of Multiple Conventional AMDAR Aircraft Observations to Diagnose the Evolution of Stability and Vertical Motions Prior to Tornado Development
Background
In an earlier AMDAR Newsletter article, Wagner and Petersen (2022) demonstrated that stability indices derived from individual AMDAR aircraft profiles of temperature, humidity and winds could be used to gauge the likelihood of deep convection and tornado development near the Dallas/Fort Worth (Texas) International Airport (DFW) on 21 October 2019. Radar sequences in Figure 8-1 show the development of the storms in the final two hours before tornado touchdown at 0158 UTC (marked by in the far right panel
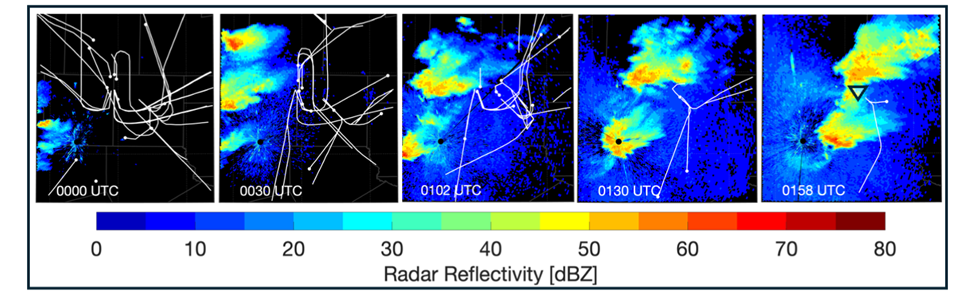
Figure 8-1: Fort Worth, TX radar reflectivity overlayed by concurrent 15-minute AMDAR flight tracks, starting at 0000 UTC, before tornado touchdown at 0158 UTC on 21 October 2019. Location of the EF3 tornado indicated in rightmost panel.
In that study, both Convective Available Potential Energy (CAPE) and Lifted Index (LI) derived only from AMDAR profiles that included moisture reports from the Water Vapor Sensing System (WVSS) showed an increasingly unstable environment from approximately 2000 UTC until 2200 UTC on 20 October 2019 after which time, the values remain relatively constant. Surface-based LI estimates (Figure 8-2a) also were calculated from the full array of all AMDAR temperature profiles combined with conventional surface observations. These stability estimates provide more temporal detail than shown previously and are available at all airports where any AMDAR profiles are observed. Although the overall trends are similar, the surface-based LIs underestimated values calculated using WVSS-II reports, especially between 2200 and 0100 UTC on 21 October 2019. In the hour immediately prior to tornadogenesis, however, the more numerous surface-based LIs showed rapid destabilization. In addition, helicity values calculated from the full set of AMDAR wind profiles revealed exponential increases in the two to three hours prior to tornado touchdown.
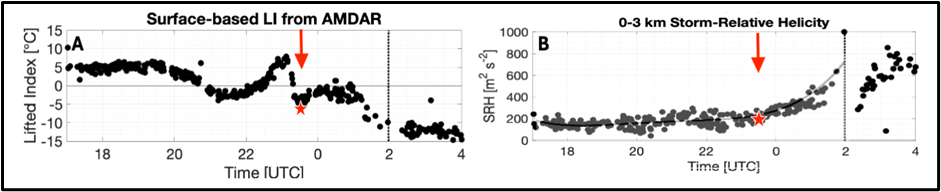
Figure 8-2: Time sequences of Surface-based LI (A) and Helicity (B) for seven hours prior and two hours after tornado touchdown near DFW at 0158 UTC 21 October 2019. Red stars indicate rawinsonde value. Trend line in grey.
Although these stability and helicity time series clearly demonstrate that AMDAR profiles can provide high-resolution forecasting information not available from other operational data sets, this note addresses an equally critical question for nowcasting severe convection – Can the amalgamation of the many real-time AMDAR wind reports in the area around DFW be used to monitor the evolution of the dynamical lifting mechanism needed to trigger convective initiation at a similar temporal resolution to complement the stability information available from individual AMDAR ascents/descents?
Methods and Results
Because vertical motion represents the sum of the divergence present between the earth’s surface and any level in the atmosphere, divergence first must be calculated for multiple layers throughout the troposphere. Figure 8-3 shows the positions of individual AMDAR aircraft landing or departing from DFW in 200 hPa layers from the surface to 400 hPa between 2200 UTC 19 October and 0130 UTC 20 October 2019 – this is the focus of this study. Not only were multiple reports present at all levels in the hours prior to tornado formation, but equally important, these reports were distributed quite regularly around the airport. These are both necessary conditions for determining reliable values of divergence and, from that, the formation, timing and strength of a local lifting mechanism.
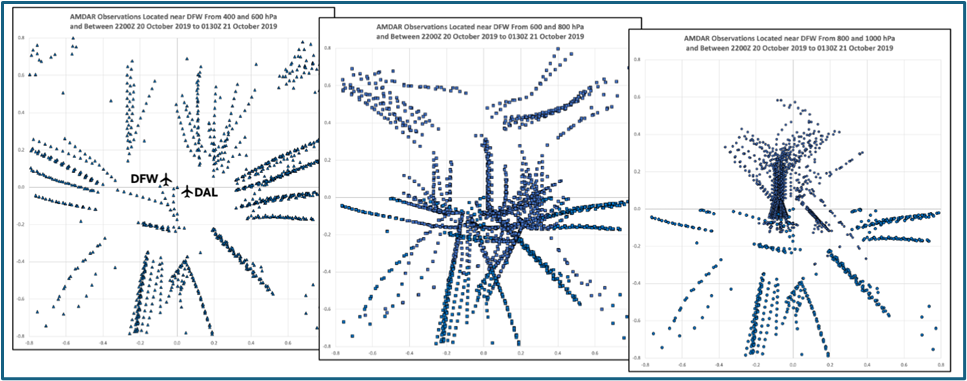
Figure 8-3: AMDAR aircraft locations relative to Fort Worth Texas in 200 hPa deep layers from 2200 UTC 20 October 2019 to 0130 UTC 21 October 2019. Distance scale is in equivalent degrees of latitude where 1o=111.111km. Top left - upper- troposphere (400-600 hPa), center - middle-troposphere (600-800 hPa), bottom right - lower-troposphere (800-1000 hPa).
Divergence was calculated using all AMDAR wind reports surrounding the airport from the surface to at least 400 hPa. First, layer averages were calculated for all AMDAR wind reports and their locations from the south, north, west and east halves of areas extending 66 km in each direction from DFW. If the reports had been distributed perfectly evenly, standard finite differencing methods then could have been used to calculate divergence from the variations in the average wind components located due east and west and due north and south of DFW. However, clustering of AMDAR reports seen along pre-specified takeoff or landing patterns prevented the necessary uniform data spacing from occurring.
An alternative method for calculating divergence was used based on the line integral approach of Helms and Hart (2013). They applied a form of Green’s theorem in which a four-sided polygon is fitted to the average locations of the observations to calculate divergence (D) using the formula:
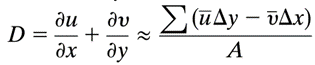
where u and v indicated average wind components between the vertices of the polygon, Dx and Dy are the lengths of the sides of the polygon and A is the area of the polygon. Wagner et al. (2022, JTech, AMS) demonstrated the validity of this method by comparing it to finite differences calculated from gridded model output.
Tests of different temporal and vertical data intervals showed that 50 hPa layers and 30-minute time periods preserved a maximum amount of detail during this event. Figure 8-4a shows both the average number of AMDAR wind reports that were available in each direction around DFW and the average minimum number of observations that were available at any of the polynomial vertices used in solving Equation 1. At all levels, an average of at least 10-20 reports were available in each quadrant around DFW. A large volume of reports helped to reduce the impact of random data errors assuring that small-scale turbulent effects and observational errors during ascent/descent maneuvers were minimized. At only a few times were fewer than four observations used in any individual layer, usually between 700 and 800 hPa, when aircraft were transitioning between rapid ascent or descent rates.
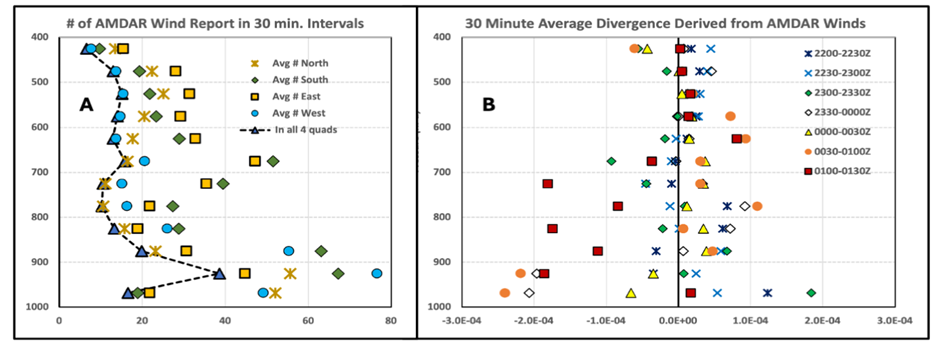
Figure 8-4: Panel A: Average number of AMDAR wind observations available for 50 hPa layers at 30-minute intervals in areas extending 66 km in each direction from DFW. Triangles and dashed line show average minimum number of observations used at any corner of the polynomial used in solving Equation 1. Panel B: Divergence calculated using AMDAR reports centered near DFW (in units of sec-1) calculated at 30-minute intervals and over 50 hPa layers from 2200 UTC through 0130 UTC on 20-21 October 2019. (Note: Divergence calculation in the bottom most layer can be degraded by a combination of observational error during maneuvers required during take-off and landing, near-surface turbulence and non-uniform observation distributions that are biased along the runway directions. To alleviate these effects, the bottom layer divergence was adjusted based on downward extrapolations of the two layers above it.)
Figure 8-4b shows divergence calculated at each 50 hPa layer and for seven successive 30-minute time periods from 2200 UTC on the 20th through 0130 UTC on the 21st. Several features stand out. First, the divergence values show good consistency both vertically and temporally throughout the period, except nearest the surface where values are especially subject to small-scale turbulence and maneuver-related aircraft observational errors (see details in Figure 8-4 caption). During the first half of the period, most layers showed small divergence at all levels with values increasing toward lower levels. Weak divergence continues at higher levels throughout the entire period. The initial lack of low-level convergence is consistent with general subsidence in the area acting to maintain the capping inversion several hours prior to storm formation even when the stability indices indicated a high risk of storm formation. After 2330 UTC, convergence appears immediately above the surface and increases over time both in magnitude and depth with values approaching -2x10-4s-1 after 0100 UTC. Continued growth of convergence directly above the boundary layer immediately prior to storm formation is essential for the development of a lifting mechanism needed to break down the capping inversion.
Vertical motion was derived from divergence by integrating the continuity equation upward from zero at the earth’s surface using the values for each 50 hPa layer and a surface elevation of 185m (~988 hPa). Profiles of vertical motion in Figure 8-5 provide quantitative evidence of this process. Results are presented in both isobaric (Panel 5a) and Cartesian (Panel 5b) coordinates.
In the earlier time periods, both panels show continuous weak subsidence at all levels indicating support for a continued lower-level capping inversion. In the 30-minute period after 2330 UTC, the picture changes substantially with the first evidence of lifting present near 900 hPa (white diamonds). This lifting, however, is short-lived and relatively shallow, limited primarily to the bottom 250 hPa of the atmosphere. From 0000 to 0030 UTC, low-level lifting has lessened as weak subsidence returns aloft.
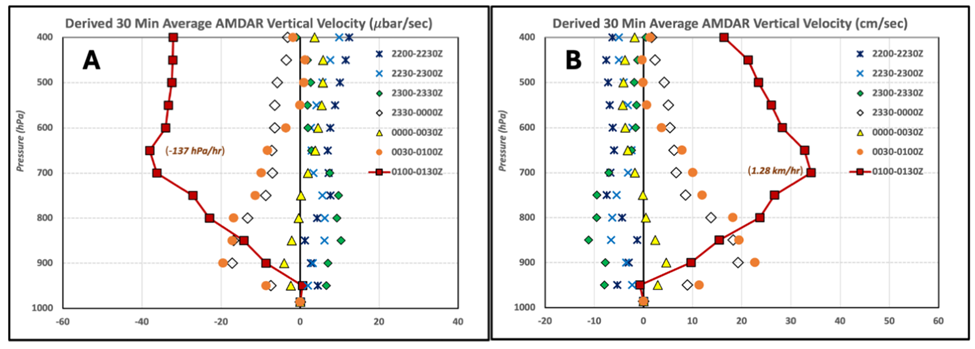
Figure 8-5: Vertical motion determined from 30-minute divergence in AMDAR winds around DFW on 20-21 October 2019. (Panel A in microbars s-1, Panel B in cm s-1). Numbers in parentheses indicate maximum hourly uplift after 0130 UTC.
Between 0030 and 0100 UTC, a second episode of low-level lifting again is detected extending above 600 hPa. This lifting is overlayed by a region of neutral vertical motion, like that of an hour earlier. However, in the next 30-minutes (ending a half hour before the tornado touchdown), the vertical motion profile around DFW suddenly shows strong ascent, exceeding 39mbar s-1 at 675 hPa and above with no subsidence aloft. On average, air parcels originating near 700 hPa are being lifted at a rate of nearly 137 hPa/hour (about 35 cm s-1 or approximately 1.23 km/hour), more than enough lift needed to release the latent instabilities. Simultaneously, the relative vorticity in the lowest 150 hPa has nearly tripled in the last half hour (not shown) coincident with the rapid increase in helicity.
Although subsidence near the surface may have contributed to skewing the surface-based LIs toward more stable values before 2200 UTC, the convergence forcing the strong upward motion at 850 hPa and 750 hPa (blue and red lines in Figure 8-6) after 2330 UTC may also contributed to moistening/warming of the surface layer and thereby increased surface-based LI values in the last two hours prior to convection.
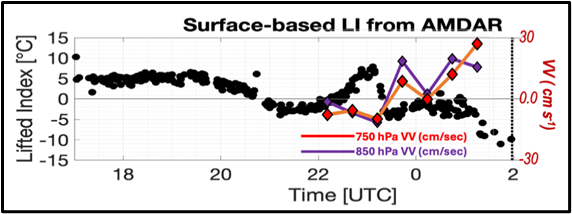
Figure 8-6: Copy of surface-based LI (from Figure 2a) superimposed with time series of 850 hPa (blue line) and 700 hPa (red line) vertical motion (cm s-1) derived from standard AMDAR wind reports.
Summary
It has been shown that the combination of surface-based LI values and helicity derived from temperature reports from all AMDAR ascents/descents at DWF provides forecasters with additional very-high-frequency information to monitor changes in stability over the airport. This greatly expands the number of airports where AMDAR profiles can be used to monitor local stability changes. Stability indices alone, however, cannot answer the critical question in nowcasting severe convection – When will the instability be released?
Real-time profiles of divergence were calculated successfully using wind observations from all AMDAR aircraft flying above and in the near vicinity of the airport. The resulting high time- and space-resolution profiles of vertical motions clearly differentiate the transition from subsidence to rapid lifting. This provides a new tool to alert forecasters as to when the energy contained in an already unstable atmosphere will be released and whether the resulting convection could produce tornadoes.
No other operational data set can provide such high-resolution (50 hPa vertical and 30-minute temporal) information about both the state and evolution of the atmosphere during the hours prior to severe thunderstorm formation, as well as the development of lifting mechanisms needed to trigger the convection. The quality, consistency and temporal resolution of the information derived from readily available operational AMDAR observations are comparable to the special data sets used in expensive and limited period research programs. Importantly, the techniques demonstrated here can be applied at any airport with a moderate number of AMDAR ascent/descent profiles without any additional cost to users, including worldwide locations where conventional rawinsonde observations may not always be available.
Ralph A. Petersen, Cooperative Institute for Meteorological Satellite Studies Space Science and Engineering Center, University of Wisconsin – Madison (ralph.petersen@ssec.wisc.edu)
Timothy J. Wagner, Cooperative Institute for Meteorological Satellite Studies Space Science and Engineering Center, University of Wisconsin – Madison
US-NWS Storm Prediction Center Uses AMDAR in Severe Weather Forecasts
AMDAR observations have been utilized increasingly in convective watches and mesoscale discussions by the NWS Storm Prediction Center (SPC) in Norman, Oklahoma, USA. They often provide the necessary environmental information needed when deciding whether to issue severe thunderstorm or tornado watches, or whether to issue forecast guidance for significant winter storms.
The SPC is one of the U.S. National Weather Service’s national centers that support NWS forecast offices in the protection of life and property. SPC meteorologists monitor the atmosphere 24 hours a day to determine where severe weather (thunderstorms, winter storms, wild fire potential) is possible over the continental United States. Numerical weather prediction models often are employed to assess where severe weather is expected. Later, when severe weather is developing, or is imminent, observational data are used to determine storm structure and impacts to people and property. AMDAR reports have become an important part of observational data alongside radar, satellite and surface observations.
AMDAR observations were used by the SPC in a data-sparse area of south Texas to determine that the atmosphere aloft was sufficiently moist and unstable for thunderstorms over Mexico to move into south Texas despite low-level stable air. This was mentioned in the mesoscale discussion during the evening hours of March 16, 2024 (see in figure 9-1).
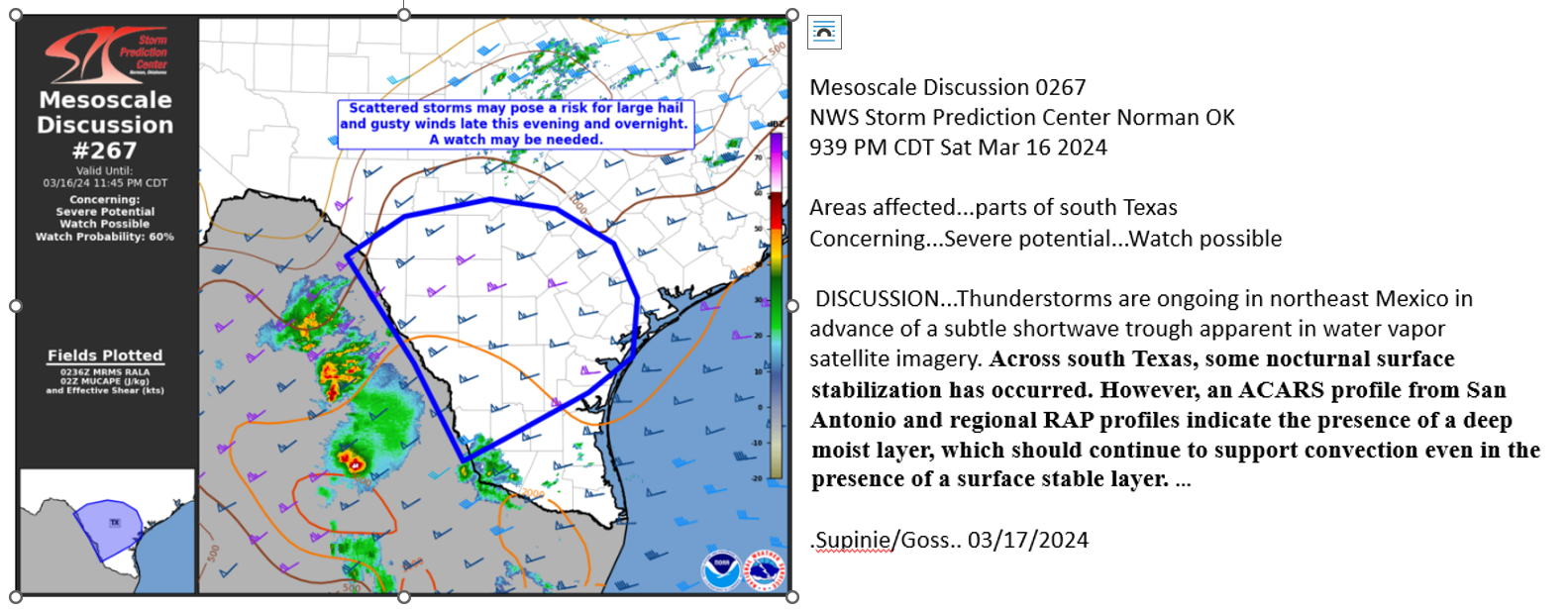
Figure 9-1. Mesoscale discussion March 16, 2024.
An AMDAR sounding was used by the SPC on the afternoon of June 7, 2022 as a cold front was moving across Ohio. The temperature data from the sounding showed steep mid-level lapse rates while the wind data suggested that speeds were sufficient for thunderstorms to organize in a line along the front. An abbreviated forecast discussion and graphic are shown below in figure 9-2.
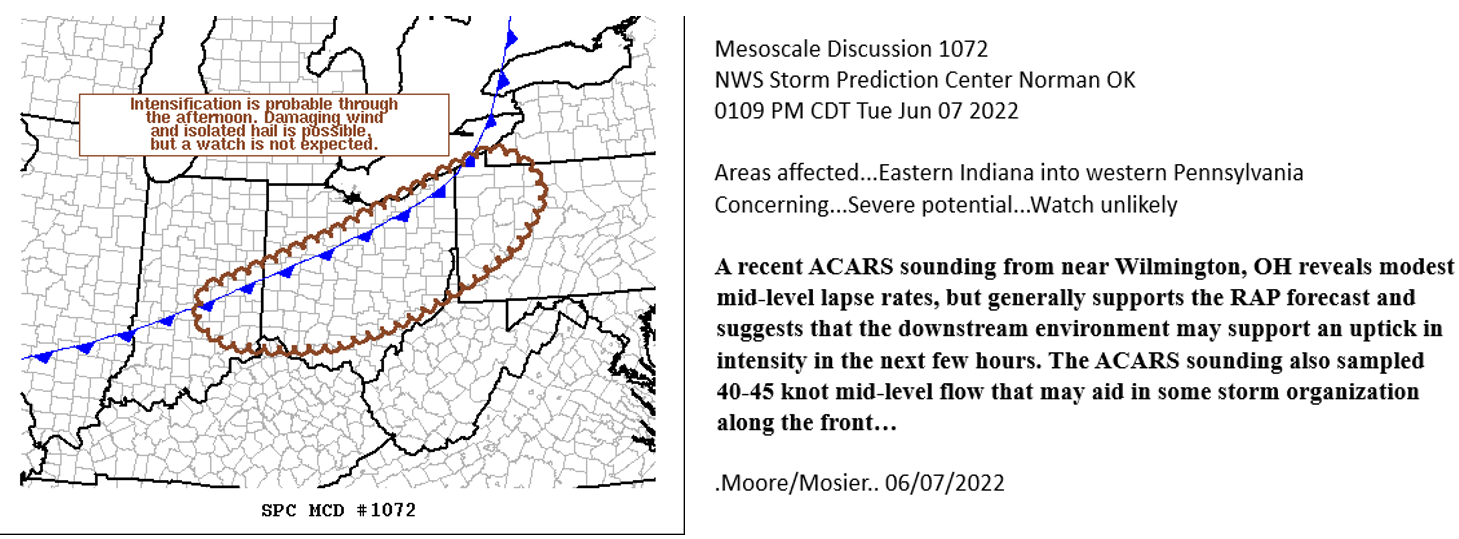
Figure 9-2. Mesoscale discussion June 7, 2022.
The SPC also finds AMDAR data useful in the winter season when issuing guidance products for winter storms. In the mesoscale discussion below (figure 9-3), SPC meteorologists noted that AMDAR soundings at Portland, Oregon continued to show dry air at the low levels. While the dry air would delay the arrival of precipitation at the surface, evaporative cooling would allow the rain to freeze upon reaching the ground. The current AMDAR soundings were particularly useful as the latest radiosonde profile from Salem, Oregon was from 12 UTC, ten hours previous.
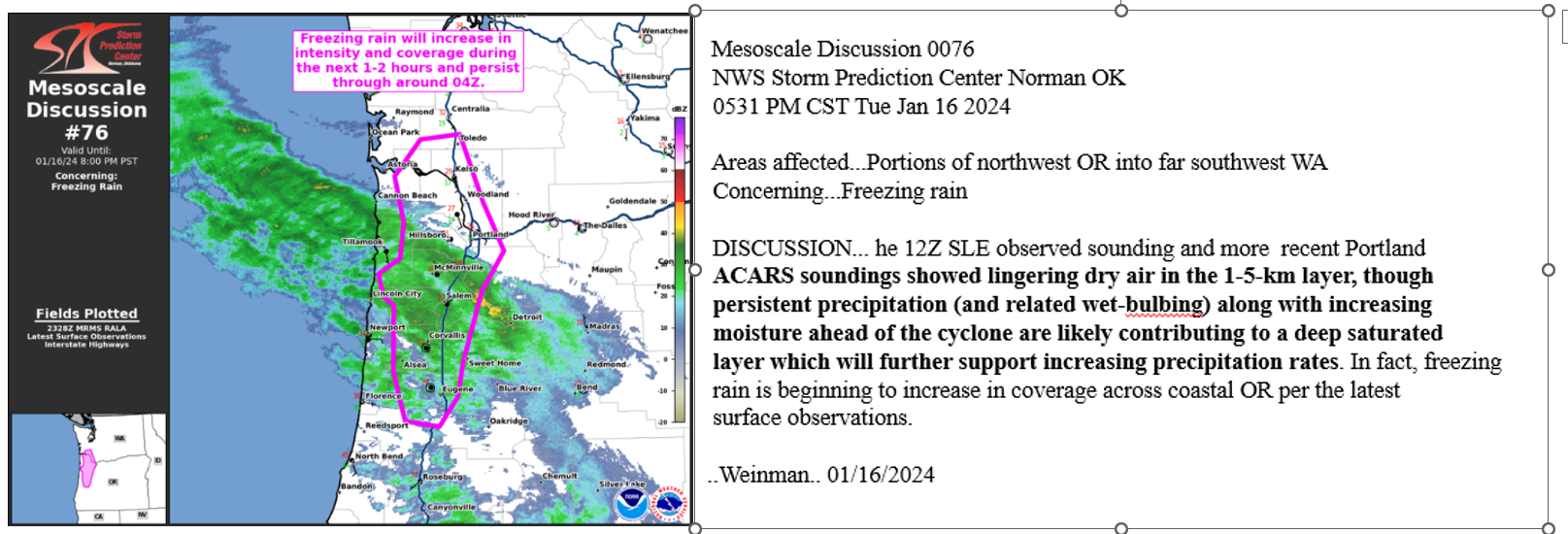
Figure 9-3. Mesoscale discussion January 16, 2024.
Richard Mamrosh, U.S. National Weather Service, Green Bay, Wisconsin, (richard.mamrosh@noaa.gov)
UAS Demonstration Campaign Status & Progress
Over the past decade, numerous technological advances in weather-sensing Uncrewed Aircraft Systems (WxUAS) have made these observing systems a reliable, accurate and environmentally friendly option for rapid in situ sensing of the lower atmosphere. Numerous reports have outlined the need for more observations in the lower atmosphere to improve the prediction of high-impact weather events (e.g., National Academies 2018, NOAA 2020). Since 2021, the UAS sub-group (UAS-SG) within WMO Expert Team on Aircraft Based Observations (ET-ABO) has been planning and coordinating a global UAS Demonstration Campaign (DC). The main goal of this campaign was to evaluate and advance the operational readiness of weather-sensing UAS to support operational meteorology through the collection of gap-filling observations and their provision to numerical weather prediction centers (WMO 2023).
The WMO UAS DC took place from 1 March through 30 Sept 2024. WxUAS were flown at more than 50 different locations and 13 countries around the world with regional concentrations in the U.S. and Europe (Figure 10-1). An assortment of different light-weight WxUAS of various sizes participated in the UAS DC (Figure 10-2). While the WMO UAS DC encouraged participants to conduct regular operations throughout the campaign, it was recognized that, due to budget constraints, it would be advantageous to target periods when simultaneous participation had the greatest likelihood. As such, three periods were chosen to serve as special operations periods (SOPs):
SOP #1: 08 April 2024 (U.S. focus, total eclipse)
SOP #2: 26 July – 11 Aug 2024 (European focus, Paris 2024 Olympics)
SOP #3: 8-13 Sept 2024 (International Society for Atmospheric Research using Remotely piloted Aircraft [ISARRA] Flight Week Field Campaign, northeastern Oklahoma, USA)
The WxUAS measured temperature, pressure, relative humidity and winds by performing vertical profiling or horizontal transects. Some UAS also collected high-rate 3D wind data that can be used to determine turbulence intensity. As of 15 October 2024, nearly 12,000 UAS data flight profiles have been collected with maximum attained altitudes ranging from 120 to 6000 m above mean sea level (MSL) (Figure 10-3). Observation files were uploaded into the Amazon Web Service (AWS) S3 data repository, converted to BUFR and made available via the WMO Information System (WIS2.0) using infrastructure and software developed by the European Centre for Medium-Range Weather Forecasts (ECMWF) and Synoptic Data Public Benefit Corporation (PBC). WxUAS data obtained during the WMO UAS DC will continue to be accepted into the AWS data repository until March 2025 as quality controls are applied and new versions of the data become available. Plans also are being developed to create a longer-term data archive.
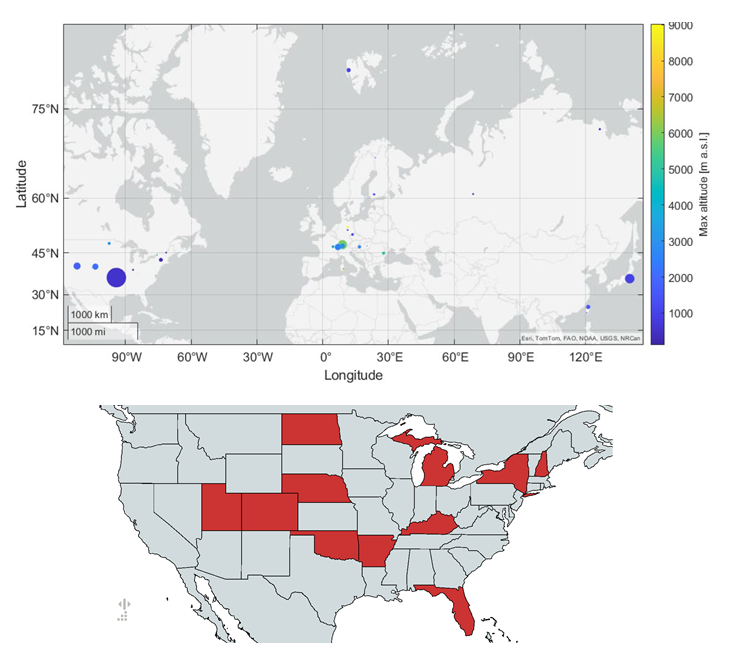
Figure 10-1: (Top) Locations of UAS observation locations with peak altitude indicated by the color and relative number of flights indicated by the size of the circles. (Bottom) States in the U.S. where UAS flights were conducted in support of the WMO UAS DC.
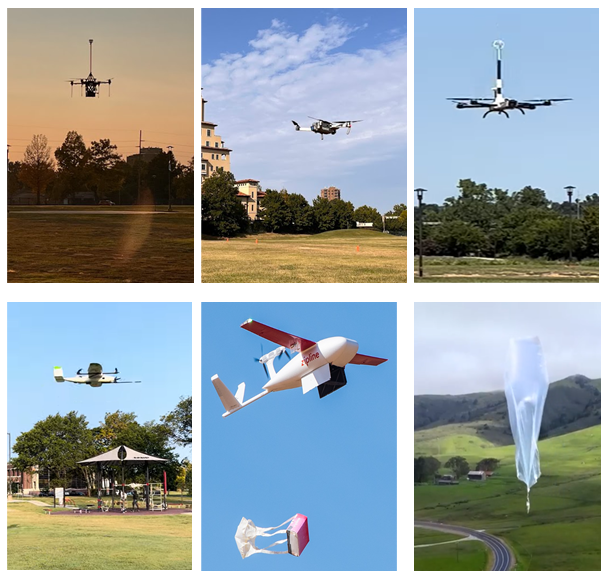
Figure 10-2: Examples of WxUAS flown during SOP#3 of the WMO UAS DC including¬ (top row) various multi-rotor UAS, (bottom-left) vertical-take-off and landing UAS, (bottom-center) observations collected and provided by UAS while performing a commercial service such as package deliveries, “observations of opportunity,” and (bottom-right) the WindBorne ballasted weather balloon.
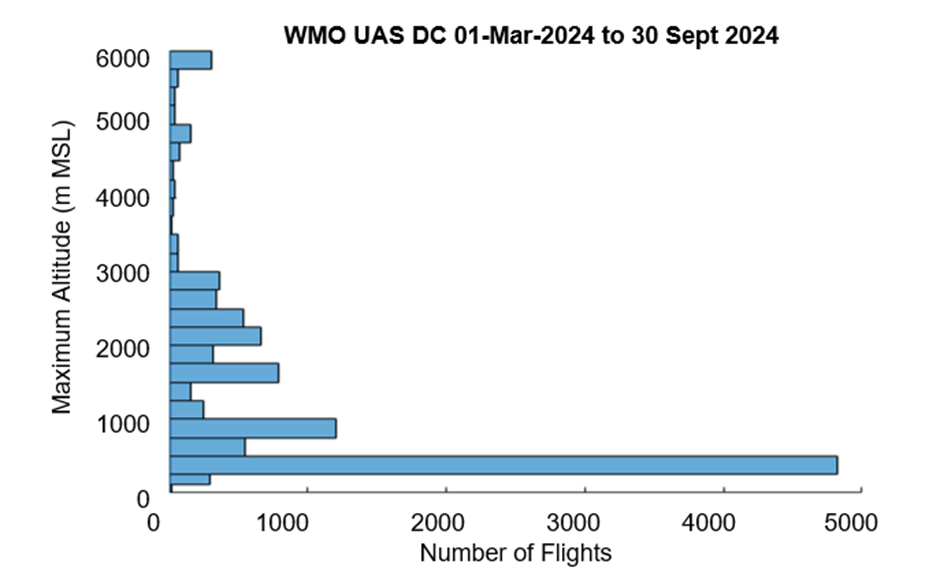
Figure 10-3: Distribution of maximum vertical height (in 250 m bins) attained by UAS during each flight. The peak count in the 250-500 m MSL bin denotes the contribution from “observations of opportunity” from Zipline delivery flights.
To support the WMO UAS DC, the WMO ET-ABO SG-UAS coordinated the participation of WxUAS activities across a range of public and private partners (private companies, universities, research laboratories) and national meteorological and hydrological services (NMHS). To achieve these goals, the WMO ET-ABO SG-UAS developed and implemented a WxUAS data format standard and teamed with ECMWF and Synoptic PBC to implement infrastructure and develop software to ingest, reformat and transmit WxUAS data in real time using an AWS S3 bucket and WIS2.0. Furthermore, WMO ET-ABO SG-UAS interfaced with civil air authorities to discuss ways of allowing WxUAS easier access to airspace. Finally, the WMO ET-ABO SG-UAS has been coordinating with modeling centers through interactions with WMO Integrated Processing and Prediction System teams (WIPPS) to quantify the impact of assimilating WxUAS observations on the skill of numerical weather prediction models.
James Pinto, NSF NCAR, (pinto@ucar.edu)
Debbie O’Sullivan, UKMET,
Maxime Hervo, MeteoSwiss,
Nicolás Rivaben, WMO, Scientific Officer, Aircraft-based Observations
Closing Thoughts
This edition of the WMO ABO Newsletter marks the 26th publication of this informative note that keeps the ABO community updated about the latest news regarding aircraft observations. As newsletter editor, I can’t thank enough the authors who have provided articles since the publication’s inception. I realize that everyone is incredibly busy and writing a newsletter article may not be their highest priority, but they still manage to find the time to make a significant contribution. I can’t adequately express my appreciation for all their dedication and effort.
The skill of the authors, especially those who have English as their second language, has made my job as editor so much easier. I know that English is not an easy language to use (I barely can speak and write it…) and the ease that all authors display has been impressive.
I would be remiss if I did not acknowledge the long-time authors who have provided articles over the years. These long-term topics include regular updates on the state of the ABO system and water vapor and turbulence measurements. Examples of data usage in operations and NWP also have been a staple of ABO newsletter editions.
It is obvious to me that the success of the WMO ABO Newsletter can be attributed to the commitment of all the authors who have provided articles over the years and I want to thank them for all their hard work.
As I look toward the future, I sincerely hope that, thanks to our authors, the WMO ABO Newsletter will continue to be an important source of ABO information.
Carl Weiss, WMO ABO Newsletter Editor
Contacts
WMO Infrastructure Commission -INFCOM- / Standing Committee on Earth Observing Systems and Monitoring Networks (SC-ON)
Expert Team on Aircraft-Based Observing Systems (ET-ABO)
Chair
Mr Curtis Marshall (USA)
Vice-chairs
Ms Carmen Emmel (Germany)
Mr Humphrey Angulu (Kenya)
WMO ABO Observing System Newsletter Editor
Mr Carl Weiss (USA)
WMO Secretariat, Aircraft-based Observations
Subscription & Links
- Newsletter email subscription - complete form & subscribe to: wmo-aircraft-observations-news
- WMO Aircraft-Based Observations
- WMO AMDAR Observing System
- Newsletters