Aviation | Hazards | Turbulence and Wind Shear
Turbulence and Wind Shear
Wind shear can be defined as ‘layers or columns of air, flowing with different velocities (i.e. speed and/or direction) to adjacent layers or columns’.
Wind shear is a major hazard for aviation especially when operating at low levels. Even when flying within a layer with a laminar flow and the flight is smooth and uneventful, the sudden crossing of the boundaries between different laminar streams will accelerate the aircraft to a greater or lesser degree. Depending on the flight direction relative to the velocity changes, shear may be felt as turbulence, but also as a sudden tail or head wind with respective consequences.
Besides convection, shear is the second major source for turbulence. Basic fluid dynamics tells us that any fluid such as the atmosphere can support only a maximum of shear between laminar flow layers before breaking down into turbulent flow.
Some aircraft are more susceptible to the effects of turbulence than others. Light aircraft are prone to be buffeted, and are significantly affected even by light turbulence. Relatively few reports of turbulence are received from fast military jets which are designed to give a high degree of tolerance.
The intensity of turbulence is categorized by the ICAO as follows:
-
Light: Effects are less than those of moderate intensity.
-
Moderate: There may be moderate changes in aircraft attitude and/or height but the aircraft remains in control at all times. Air speed variations are usually small. Changes in accelerometer readings of 0.5-1.0g at the aircraft’s centre of gravity. Occupants feel strain against seat belts. There is difficulty in walking. Loose objects move about.
-
Severe: Abrupt changes in aircraft attitude and/or height. The aircraft may be out of control for short periods. Air speed variations are usually large. Changes in accelerometer readings greater than 1.0 g at the aircraft’s centre of gravity (but note, Military aviators regard +4g/2g as severe. Objects are forced violently against seat belts. Loose objects are tossed about.
-
Extreme: Effects are more pronounced than for severe intensity.
From the above, we note that the only criterion that is not subjective is that of airborne accelerometer readings. Converting the ‘standard’ parameters available to forecasters such as wind speed, gusts, stability etc., to such values would necessarily be very difficult and would require a specific calculation for each aircraft separately. Bench forecasters, therefore, have to largely rely upon more general, empirical rules and relationships.
Wind shear, of itself, is not categorized in the same way, although when it ultimately makes its presence felt, the above turbulence categories may become applicable. Turbulence can manifest through:
- convective turbulence (i.e. turbulence due to convection)
- mechanical turbulence, mostly low-level turbulence
- orographically induced turbulence
- clear air turbulence (CAT)
- low-level jets
- wake turbulence/wake vortices
The following provides an overview of each of these forms of turbulence.
Convective Turbulence
Turbulence is always associated with convection, so for that reason this type is referred to as convective turbulence. The origin and physical cause may vary:
-
The vertical currents within and around convective clouds are turbulent.
-
Growing convective towers may generate gravity waves which propagate either radially away, for instance within the anvil, or may also propagate vertically.
-
Dry thermals (i.e. non-saturated ascending air).
-
Downdraughts associated with precipitation or mid-level cold layers of air. These can produce squall lines near showers.
Thermal turbulence over land has a marked diurnal variation, with a maximum during the afternoon and a minimum overnight. Thunderstorms, in contrast, may last the whole night and propagate over large distances of several hundred kilometres.
At its simplest, convective turbulence will result in ‘bumpiness’ in flight. Of course, as the intensity of turbulence increases, its effect will increase in accordance with the ICAO categories. Ultimately, depending on aircraft type, severe turbulence may cause structural damage to an aircraft. Airlines are most concerned with injuries to passengers which may lead to costly compensation claims. One should also note that updraught speed usually varies strongly across an updraught. Thus an aircraft flying through a convective updraught will feel not only the convective turbulence within the cloud, but also the acceleration due to the varying vertical wind speed along its cloud transect. Usually we find in a thunderstorm updraught even more hazards such as hail, lightning, heavy rain and icing.
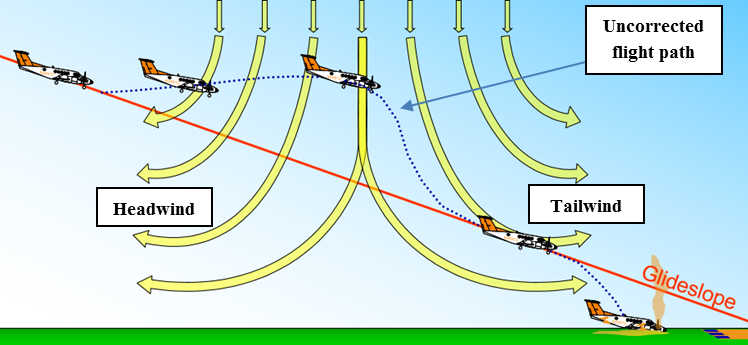
Additionally, in association with large storms, strong downdraughts or microbursts can occur producing a violent outflow of air which spreads outward on hitting the ground. Those downdraughts usually are caused by cool air sinking in the surrounding rising warmer updraught air. The lower temperatures might be accentuated by evaporating precipitation, visible as virga. Though downdraughts originate very often from deep in the cloud, the associated risk is highest below cloud base. Here we find not only a negative vertical wind speed, which by itself pushes the aircraft down; we also observe significant horizontal wind shear. The downdraught forces the air close to the ground to spread radially outwards.
Mechanical Turbulence
Mechanical turbulence results solely from shear. The latter is always found close to the surface where wind speed vanishes. Within the boundary layer and typically at night a low-level jet may be found, which also might produce turbulence. Furthermore, turbulence may also be found close to the edge of the jet stream at tropopause heights.
Close to the ground, mechanical turbulence is also often referred to as low-level turbulence. Surface friction is the primary cause of the vanishing wind at the surface. Thus the intensity of mechanical turbulence depends upon:
-
wind strength;
-
terrain roughness; and
-
atmospheric stability near the surface.
In general, the stronger the wind and the rougher the terrain, the more intense the turbulence experienced. Light winds over a smooth sea give the least turbulence.
The higher the lapse rate, the more readily vertical gusts develop and thus the more vigorous the turbulence is. In more stable air, vertical eddies are suppressed and turbulence is damped – but very stable air and a sufficient displacement over large obstacles (hills/mountains) may lead to mountain or lee wave development.
At its simplest, mechanical turbulence will result in ‘bumpiness’ in flight. The intensity of turbulence will increase in accordance with the above-mentioned criteria and flight speed. For any given intensity of turbulence, the faster the aircraft flies, the more it will be accelerated. The closer it is to the ground, the less time there is available to react to those accelerations. Ultimately, depending on aircraft type, severe turbulence may cause structural damage to an aircraft, especially when combined with inadequate, strong rudder movements.
Orographic Turbulence
If surface roughness increases and characteristic roughness heights increase as well, e.g. over cities, forests, small hills and larger hills, and mountains, the airflow suffers large corresponding displacements from its original level. Dependent upon the stability of the air mass, this may result in triggering convection, with its attendant turbulence; it also may generate gravity waves, referred to in that case as mountain waves, or may tend to return the airflow to its original level giving ‘standing waves’ and rotors. Orographic structure might be arbitrary complex and so is the associated flow pattern. One finds the airflow funnelled along valleys creating marked deviations from what might be expected from the undisturbed ‘gradient wind’, one might find blocking of the flow by mountains or hills, one might also found increased turbulence close to the ridges.
Mountain waves are generated by a flow across the mountains and can develop in stable atmospheric conditions. These wave motions may persist for hundreds of kilometers downstream:
-
in warm sectors;
-
in strong winds around the periphery of anticyclones; and
-
ahead of warm fronts.
Mountain waves are nothing more than gravity waves and therefore we can classify them as ‘trapped’ or ‘untrapped’.
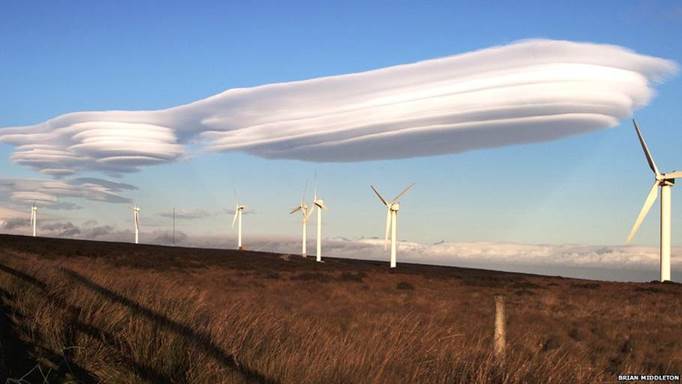
Mountain waves can be both an advantage and a disadvantage to aviation, mostly however the latter is the case.
Experienced glider pilots look for the updraught side of mountain waves in order to gain altitude. With ascent rates of around 500 ft per minute they can be very useful in gaining height quickly. Within such updraughts the flight may well be very smooth.
There are several inherent dangers:
-
The rapid change in height can mean that a pilot caught unawares may very quickly conflict with aircraft at different flight levels, and more importantly, if caught in a downdraught may rapidly erode any terrain clearance margins, and ultimately cause impact with the ground. Such effects will be most pronounced if the aircraft track is parallel with the ridge. Mountain wave activity is noted on aviation charts when vertical velocities reach and exceed 500 ft per minute – the maximum climb rate of some models of Cessna light aircraft are of the order of 700 ft per minute. Clearly, higher powered commercial and military aircraft will normally be able to climb more rapidly, but it does give an indication of how important a 500 ft per minute downdraught can be to the pilots of light aircraft.
-
The laminar and smooth flow will break down to give rotors in the crests of the first one or two lower level waves of the flow – turbulence should be expected to be severe in these regions, and may or may not be marked with ‘roll cloud’.
-
If the wavelength is short, then an aircraft travelling swiftly through and perpendicular to the wave-train will experience a prolonged series of rapid fluctuations of vertical velocity. This will result in turbulent flight.
-
Turbulence may be experienced in association with mountain wave motions, particularly if the vertical currents are strong and the wave length is short. Turbulence-prone areas are most likely to be near wave crests and troughs, while at mid-levels, the flow may be quite smooth and laminar. As with all gravity waves, mountain waves may also break causing then severe turbulence.
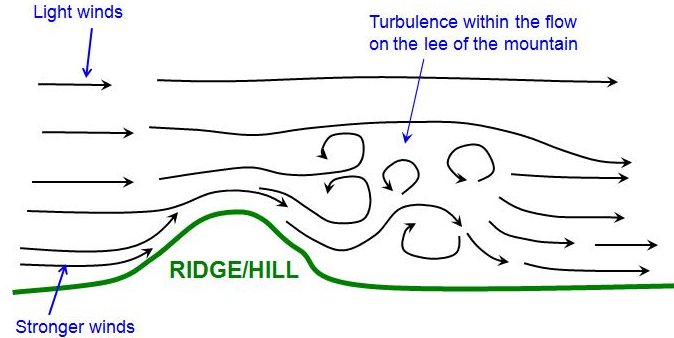
Surface rotors are extremely hazardous to aircraft. Aircraft may simply not be able to stabilise their approach. Not only may wind direction change abruptly (wind shear) causing marked changes in lift and drift, but the aircraft also may be affected by strong updraughts and downdraughts. The turbulent flow can quickly be replaced by the very strong flow of air on the lee-side, often well outside cross wind limits of aircraft. It is possible for windsocks at different locations within the perimeter of an airfield to all indicate markedly different wind directions and strengths.
Clear Air Turbulence
Clear air turbulence (CAT) is the term used to describe medium- or high-level atmospheric turbulence produced in regions of marked wind shear. As its name suggests, this often though not necessarily always occurs in the absence of cloud, making it difficult to detect visually.
As with all turbulence types, the degree of turbulence is categorized by the ICAO definitions. In extremes, structural damage to the aircraft may occur.
For civil aviation, passengers may be made uncomfortable, or suffer injuries when not wearing their seat belts. Fatalities have occurred as a result of turbulence encounters.
Low-level Jets
There are several forms of low level jet stream. One form can be described as a tube of enhanced low-level wind flow along and ahead of a cold front. As such, it is associated with the front, and will move with it.
Another form of low level jet can be described as increased wind flow caused by the formation of a nocturnal inversion and associated decoupling of the gradient and surface wind regime. When the flow is decoupled, the surface flow becomes very much lighter, but the flow immediately above the discontinuity is no longer affected so much by friction (or viscous drag), and so becomes stronger.
The nocturnal lowlevel jet (or wind maximum) is a boundary layer feature at heights between 50 and 1000 m. It may be confined to a shallow layer of a few decametres deep. Shear may be significant with values of 20 m/s per 50 m. The nocturnal lowlevel jet is a typical inertial flow occurring under weak pressure gradients. The wind direction turns during the night according to the inertial frequency 2π/f. Due to the shear at the lower and upper part of the jet, turbulence may be generated there and/or gravity waves may be excited. Nocturnal low-level jets are a frequent feature in certain areas of the world, especially over the lower plains of Australia, of Northern Central Europe and over the Great Plains in North America.
There is a type of low level jet, referred to as a ‘sting jet’, that can form around low centres during explosive cyclogenesis. Such jets are regions of enhanced wind flow, caused by descending air accelerating as it is cooled (and therefore becoming denser) due to precipitation evaporating as the precipitation falls through the already descending air. It should be noted that the ‘sting jet’ is still being studied, and its precise method of formation will be more complex than the simple description above.
An example of a ‘climatological’ low level jet would be the seasonal development and subsequent decay of the ‘Somali Jet’. The Somali Jet is a feature of the northern hemisphere summer, and the development of the Asian Monsoon. When fully developed a southeasterly low-level flow crosses the northern tip of Madagascar, before veering southerly and then southwesterly across the Horn of Africa to become a southwesterly flow running parallel to the coasts of Yemen and Oman. The jet has important climatological effects, but with maximum winds of some 40 or 50 kt at the 850 hPa level, there is an aviation aspect to consider.
Turbulence effects on the low-level cold front jet can be marked, and unexpected. Wind shear across the boundaries of the jet will need corrective action on the part of the pilot. In concrete terms, the low-level jet implies that terrain clearance may be compromised, and difficulties during the landing phase may be encountered.
Nocturnal jets may not have any apparent turbulence associated with them whilst the pilot remains on one side of the discontinuity. Crossing the boundary may result in a period of turbulence, but it is the change of airflow across the wings at low level that may adversely affect lift – especially in the landing and take-off phase.
Wake Turbulence
Wake turbulence is a result of the vortices formed in the wake of aircraft. Vortices form on the top surface of each wing, and are left in the aircraft’s wake. Helicopters also produce wake turbulence, with vortices generated from the main rotor blades.
In their formation, they are not of ‘meteorological’ origin as they serve to keep the aircraft flying and transport momentum downwards. Primarily, they are a function of the weight, size, and aerodynamic properties of the aircraft. However, once formed, they are known to sink with a speed of 12 m/s, and will be transported with the general wind flow.
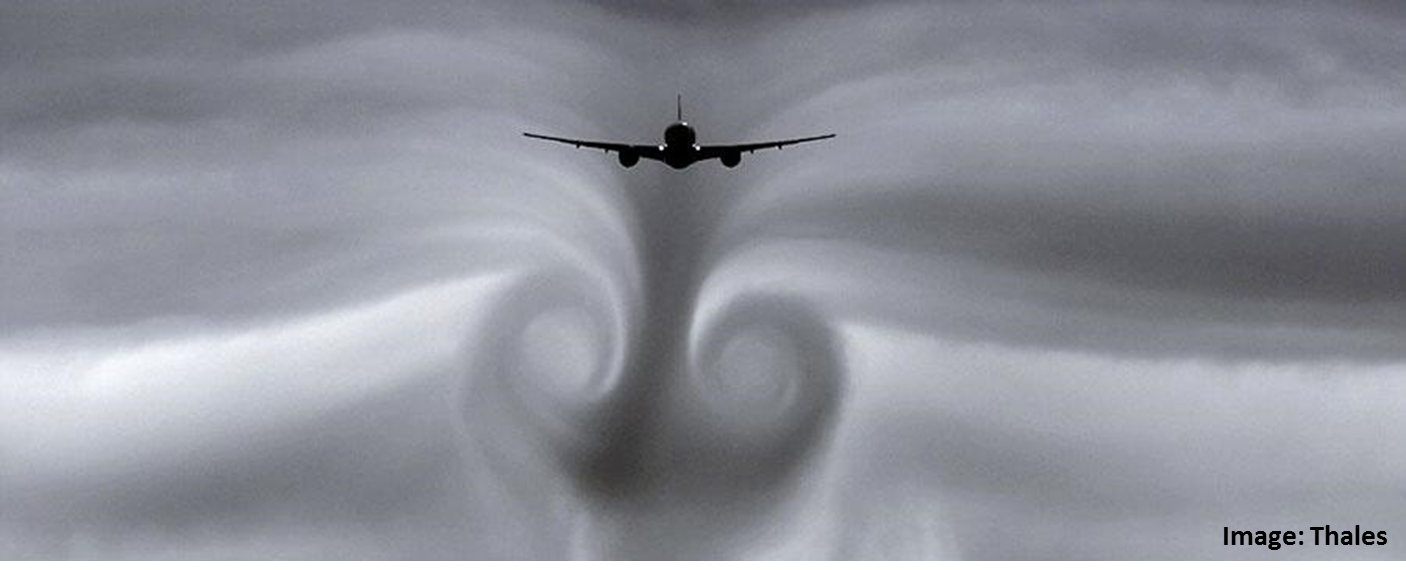
As with all forms of turbulence, encounters at low level can prove fatal, with little room or time for recovery. It might be considered that the worst case scenario would be that of two aircraft taking off. All other things being equal, the lead aircraft is likely to be full, in terms of passengers, cargo and fuel. Because of its weight it will generate more intense wake vortices/turbulence. The second aircraft takes off, and it too will likely be full and heavy. On encountering the turbulence, because of its weight the second aircraft will be less responsive to control input and engine power settings, and may well impact the ground.
Light aircraft that encounter the wake turbulence of heavy airliners may be violently tossed around.
Even after takeoff, aircraft can encounter wake turbulence unexpectedly. Consider an aircraft taking off in a northward direction, and departing the area. If the wind is from the west, then the wake will drift eastward. A second aircraft may take off, turn towards the west, and subsequently catch up with and encounter the previous aircraft’s wake.
The simplest precaution for pilots is to remain a safe distance behind the leading aircraft. A light aircraft must remain at a greater distance behind a heavy aircraft than the distance that a heavy aircraft must remain behind a similar heavy aircraft. Currently, it is the responsibility of Air Traffic Control and the pilot in command of the following aircraft to maintain such separation and to comply with prescribed internationally agreed separation distances. To increase airspace capacity on the ground and in the air, there is a growing demand within the aviation industry to reduce such separations to a still safe minimum, and meteorology will play a part in this.
Contrails forming at the exit of aircraft engines will be incorporated in the wing tip vortices. Thus, from a four engine aircraft, only two separate contrails remain beyond one aircraft length behind. Subsequently the behaviour of the wake vortices may be monitored by the behaviour of the contrails. Usually, wake vortices exhibit a longitudinal variation which finally leads to a break-up of the vortices. This break-up is enhanced, and occurs earlier, if ambient turbulence is high.
CLICK HERE to return to our homepage